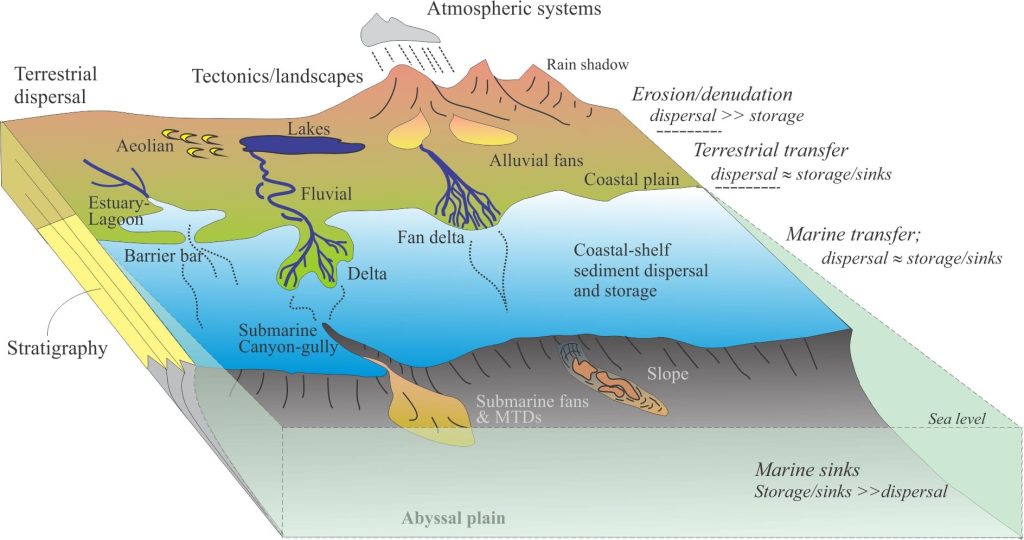
Sediment routing systems – or how sediment is dispersed between source and sink
Earth scientists routinely talk about the rock record, the rocks we see, touch, or image, that exist because of a multitude of physical, chemical, and biological processes. In one sense, they represent a kind of endgame, a closing statement that records Earth’s propositions. Thus, a deep sea turbidite, shelf cycle, or fluvial point bar represents the end-product of multifarious depositional processes, framed in the context of tectonics, climate, sedimentary basin dynamics (uplift, subsidence), fluctuating sea levels, past lives, and provenance that tells us whence the clastic material was derived.
But neither provenance nor the analysis of sedimentary facies will tell us much, if anything, about the actual journey each sedimentary particle took, from its inception at some distant source to its final resting place. Deciphering this journey, or sediment routing, would tell us a great deal about the dynamic relationship between drainage basins (sediment sources) and down-stream sedimentary basins (sediment sinks).
The sediment cascade from source to sink has come under a great deal of scrutiny over the last couple of decades. Geomorphic analysis of modern drainage basins in terms of climate, relief, size, and longevity, combined with numerical modelling of denudation rates, sediment flux and dispersal, has greatly improved our understanding of sediment routing systems.
The geomorphic components of sediment routing systems
Stanley Schumm (1977) provided a useful framework for unraveling sediment routing based primarily on his work on terrestrial depositional systems, by dividing them into zones of erosion/denudation (the source region where sediment is produced; also called the erosion engine), sediment transfer zone, and sediment deposition (the sink) – each expresses a relationship between sediment flux (or mass transfer) and sediment storage. A more recent statement by P.A. Allen (2017, Chapter 1) that encapsulates these ideas reads; “Sediment routing systems link the fate of particulate sediment from source to sink, and essentially frame the problems of denudation, sediment transport and deposition as a box model characterised by sources, reservoirs and sinks with connecting fluxes”.
Nyberg et al’s. (2018, open access) description of these systems is a bit more specific: “Source-to-sink systems comprise adjacent linked segments, commonly hinterland catchments, alluvial- and coastal plains, the continental shelf, continental slope and submarine fan”.
Let’s unravel these statements:
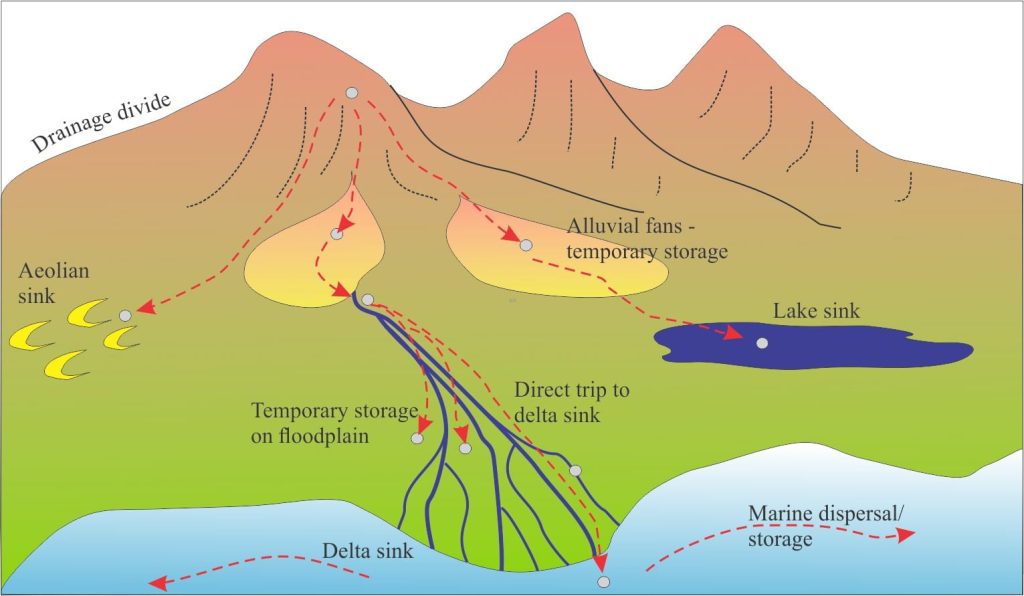
- The sediment routing system follows the actual paths that clastic material takes from source to sink. Paths may be direct or circuitous.
- The erosion engine: The top of a routing system is defined by a source area, usually of high relief, where particulate sediment and dissolved solutes are formed, primarily from regoliths (chemical and physical weathering), that are subjected to erosion. Mass wasting by slope failure (e.g., landslides, rockfalls) also plays a role. Denudation may be initiated by tectonism (e.g., foreland thrusting) combined with an isostatic response (uplift) that depends on the rate and extent of erosion. The source area is a region of net denudation; sediment storage is mostly temporary and prone to reworking (for example thin sheets of colluvium, or talus cones).
- Transfer zone: The cascade of sediment across the terrestrial transfer zone occurs via many processes – fluvial and alluvial transport (channel, overbank, sheetflood), debris flows and lahars, and aeolian processes (Allen and Allen, 2013). Marine environments also participate in sediment transfer zone, where tidal transport, wave action and submarine slope failure move sediment across intertidal, shelf and slope environments. Sediment may also bypass these marine realms during sea level lowstands, as fluvial and aeolian processes follow the receding shorelines, sending sediment through shelf-break gullies and submarine canyons, across the continental slope to the deep basin beyond.
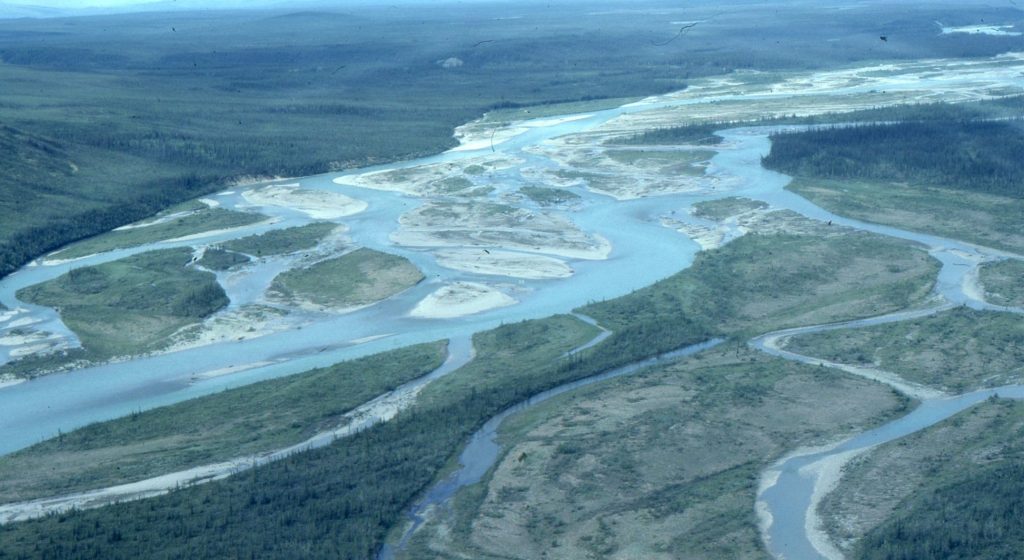
Overall, there is a balance between sediment transfer and storage, but at any point in time the balance may be tipped by perturbations in supply promoted by tectonics, or eustatic lowering/rising sea levels (i.e., river baselevels).
The transfer zone is perhaps one of the most challenging to unravel. Sediment may move directly to its sink (e.g., delta, or deep-water submarine fan), with only temporary stops along the way. This might be the case for narrow zones with steep gradients, bound by mountainous source terrains and narrow shelves seaward. In this situation the potential for storage will depend on the potential for accommodation over the long term, versus the propensity for high energy sediment reworking. Much broader transfer zones, on the scale of the Mississippi (3.2 x 106 km2) or Mackenzie River (106 km2) drainage basins may allow the development of both temporary and longer-term storage in fluvial sinks such as flood plains, abandoned channels and lakes.
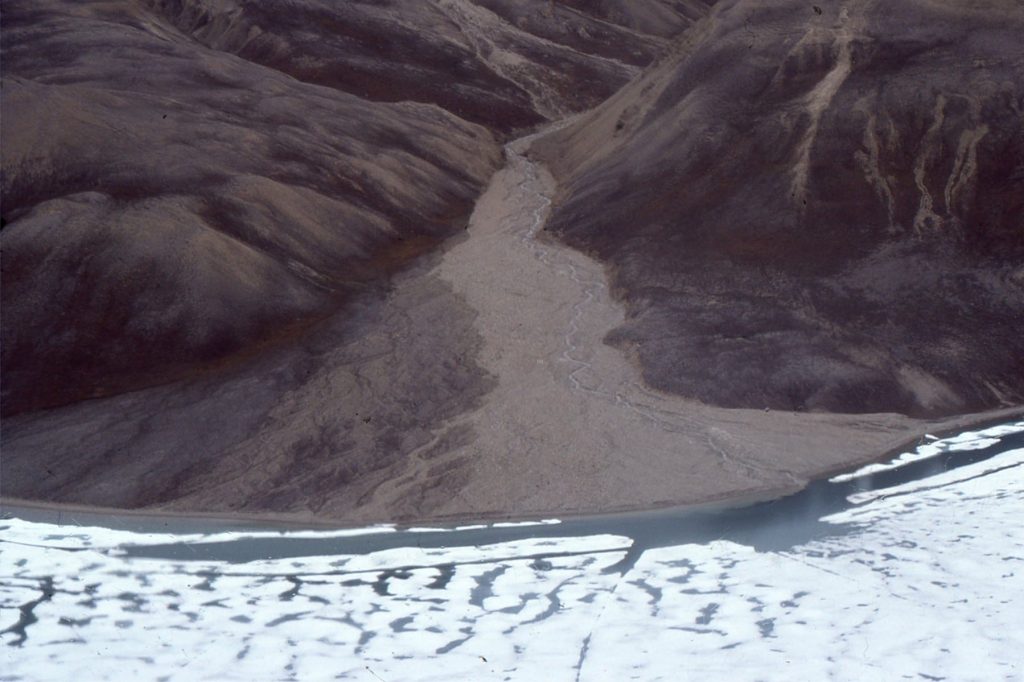
There is a general expectation, based on reasonable empirical evidence, that grain size will be fractionated within individual depositional systems (e.g., alluvial fans) and at much larger scales between source and sink (Allen et al., 2016, PDF available).
At one end of the clast size spectrum, blocky and gravelly material will tend to occur close to the source area. As the sediment load cascades downstream, hydraulic sorting and abrasion reduce the average grain size. This trend in grain size makes sense when applied to a simple, single source drainage basin and transfer zone, but many large sediment routing systems have multiple source areas that deliver sediment via tributaries at different downstream exit points. The resulting grain populations and grain compositions will reflect these different sources, the differences in rock-mechanical strength, and their textural properties (e.g., grain size, angularity, and sorting of subpopulations). One extreme of grain size fractionation lies in the production of mud (clay plus silt) that may quickly bypass the transfer zone as a suspension load, ahead of the coarser sediment cascade, although some may be trapped in lakes and river floodplains.
4. The depositional sink: At its most basic, a depositional sink is a region of final deposition – the endpoint of a sediment routing system. To be a fully functional sink, there must be net deposition, which means there must be sufficient long-term accommodation, notwithstanding the vagaries of baselevel change. Good examples of end-point deposition are submarine fans, where the transfer zone includes sediment delivery from terrestrial and shallow marine systems, through focal points defined by shelf-edge gullies and submarine canyons. We can confidently assume that the submarine fan is an endpoint because there is nowhere else for its sediment to go.
But sediment en route to the submarine fan may be stored in other terrestrial and marine sinks, some temporary, others more permanent (Nyberg et al. 2018, open access). We know this because of all those preserved alluvial, fluvial, and shallow marine shelf successions we map in outcrop or the subsurface. So, the concept of a depositional sink should be qualified thus – it is the endpoint for deposition anywhere in a sediment routing system where there is sufficient accommodation to enable its preservation. This is consistent with the idea that a there exists a balance between net sediment removal and net deposition in transfer zones.
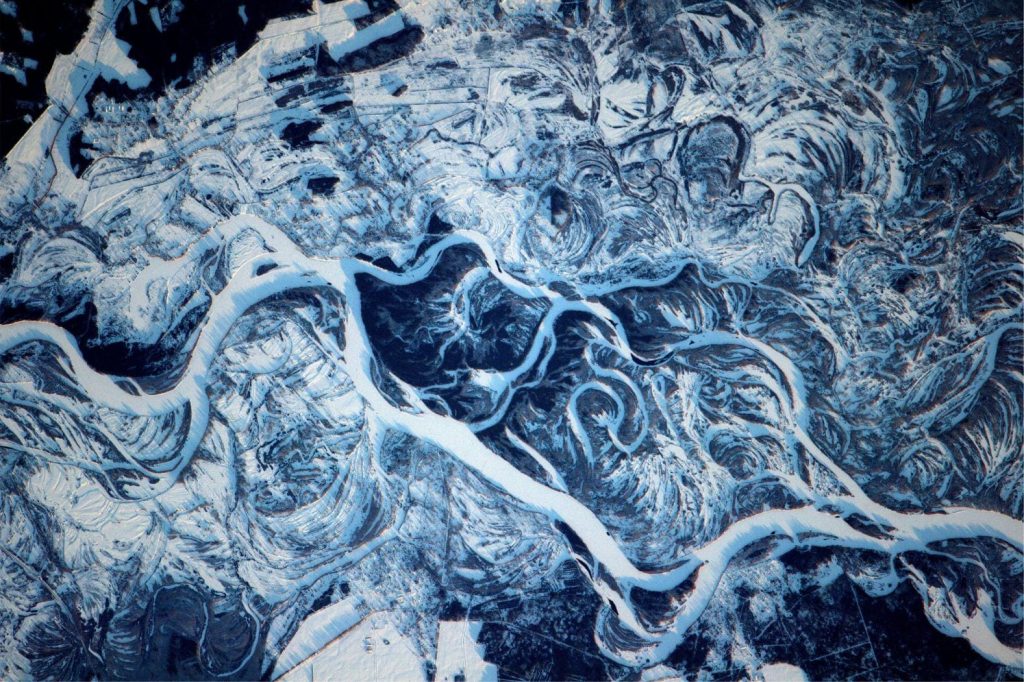
Deltas are another interesting example. They develop where the flux of river-derived sediment keeps pace with (aggradational) or exceeds (progradational) baselevel rise – in other words, where there is lots of accommodation. They are permanent endpoints for terrestrial supply routes but may also shed material to the deeper shelf and submarine fans. So, they act both as a sink, and as part of the transfer zone for deeper water depositional systems.
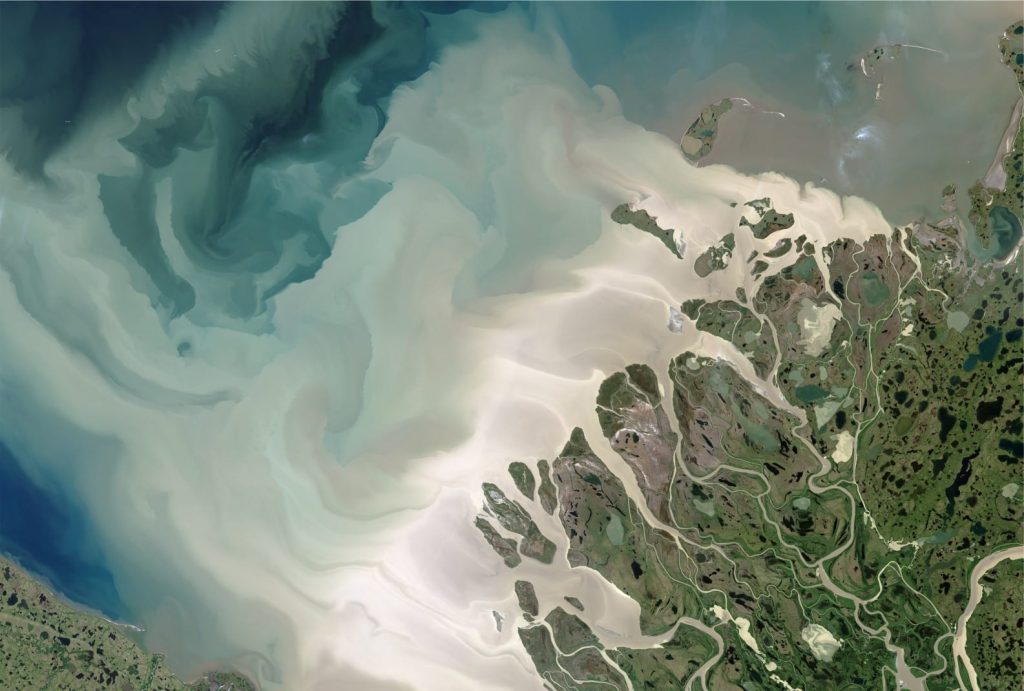
A holistic approach
Unraveling sediment routing systems is a challenge even in modern settings. It is a scientific endeavour that encompasses many disciplines – an understanding of the relationships between landscapes and tectonics, tectonics and climate, atmospheric forcing associated with erosion and sediment flux, oceanographic processes and depositional systems, the stratigraphic consequences of baselevel fluctuations, and the feedback processes that affect all of the above. Solving source to sink problems in the rock record will lean heavily on this actualistic approach.
Some of the directions this science is taking include:
- The relationship among landscapes (geomorphology), tectonics, climate, and oceanographic processes (Allen, 2008, PDF available). For example, how does climate influence the rate of isostatic uplift and subsequent denudation?
- An actualistic approach to sediment flux in modern watersheds (rates of erosion, denudation and supply), and the processes involved in its transfer to downstream sinks (Caracciolo et al., 2021);
- Estimates of sediment and solute production under different climatic and geomorphic conditions, and the mass balance between source and sink;
- Sediment flux in relation to the residence times for sediment storage en route to sinks (Romans et al. 2016, PDF available). For example, how representative are measurements and observations of historical processes in the context of, say, Milankovitch cyclicity (105 years), or at the scale of 3rd-order stratigraphic sequences (106 years)? A broader question might be – How do we scale actualistic processes (i.e., those we measure today) over deep time?
- Quantifying grain size fractionation (i.e., grain size diminution) between source and depositional sink; within watersheds, and the potential for mixing of grain size populations from different watersheds (Allen et al. 2016, PDF available).
- Analogue (e.g., sand box experiments) and numerical modelling of sediment flux and dispersal in terms of the environmental and process variables noted above, and the attendant problems of scaling those physical and rate variables (e.g., Heller et al. 2001, PDF available; Paola et al., 2009, Paola and Leeder, 2011.
Other posts in this series
Sedimentary basins: Regions of prolonged subsidence
The rheology of the lithosphere
Isostasy: A lithospheric balancing act
The thermal structure of the lithosphere
Classification of sedimentary basins
Stretching the lithosphere: Rift basins
Nascent, conjugate passive margins
Thrust faults: Some common terminology
Basins formed by lithospheric flexure
Basins formed by strike-slip tectonics
Allochthonous terranes: suspect and exotic
Geohistory 1: Accounting for basin subsidence
Geohistory 2: Backstripping tectonic subsidence