Slump folds, slides, convoluted laminae, enterolithic folds, normal and thrust faults, glide planes, fractures, boudinage, ball-and-pillow, load casts, dewatering pipes, dish structures, sedimentary dykes, seismites, liquefaction, fluidization.
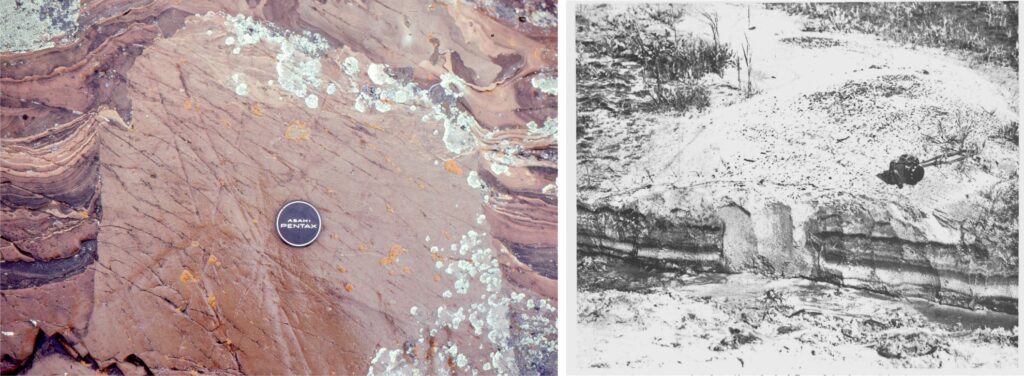
This post is part of the Lithofacies Series.
See the companion post – Rheology of soft sediment deformation
The phrase “soft sediment deformation” (SSD) has been foisted upon Earth scientists as a kind of penance. It is one of the most nebulous phrases in geological description and yet is deeply embedded in our vocabulary. Its nebulosity centres on the meaning of the word “soft” and its unspoken antonym “hard”. Also problematic are the implied conditions of timing of deformation, whether it took place during sedimentation or at some time during burial, and whether we can distinguish this deformation from tectonic processes that may be coeval or imposed much later.
Tectonic overprinting is one of the more serious problems associated with any discussion of soft sediment deformation. Many of the structures we typically associate with hard rock deformation (HRD) – folds, faults and fractures, structures formed by shear or extension (e.g., boudinage), and penetrative deformation (e.g., cleavage) can also be generated by deformation of soft sediment. Rheological conditions such as brittle and ductile behaviour can also be applied to both types of deformation. Thus, any consideration of what constitutes soft sediment deformation must also provide a cogent distinction from tectonic deformation – not a simple task because we know that both types can occur contemporaneously and in the same space, particularly at plate boundaries (e.g., Elliot and Williams, 1988).
An additional complication arises from our attempts to simplify descriptions of natural deformation by imposing a bipartite hierarchy – soft sediment and hard rock. However, we know that consolidation, compaction, and lithification (that includes diagenetic changes) occupy a spectrum of the variables that measure how ‘hard’ a rock is, notably rock strength and cohesiveness (as units of stress). There is a very broad region of rock strengths between soft (squishy) and hard that Sporli and Rowland (2007) refer to as the often-neglected weak rock domain.
Definitions and other statements
Deformation of non-lithified sediment has for decades been recognized as a process contemporaneous (or nearly so) with sedimentation in modern (actualistic) and ancient successions. Many older, now iconic texts on sedimentology used the term contemporaneous deformation for structures like slump folds and faults, glide planes, dewatering pipes and sheets, sedimentary dykes, and boudinage (F. Pettijohn (1949), W. H. Twenhofel (1950); C. Conybeare and K. Crook (1968); Reineck and Singh, 1973; J.R.L. Allen, 1982, 1985). The dominant force driving deformation is gravity – gravity induced slope failure, sliding, extension and compression; all these mechanisms can occur in the same deformed package. Differential compaction is also important where fluid over-pressures can result in focused fluid flow of the kind responsible for dewatering pipes and dykes, or liquefaction and fluidization where cohesive and shear strengths are reduced to almost zero. Differential compaction and fluid escape can occur over broad segments of sedimentary basins and in single beds, for example in turbidites where grain size grading creates permeability gradients.
C. Conybeare and K. Crook (1968) in their manual of Sedimentary Structures refer to structures formed by deformation of cohesive sediment as rheotropic structures. They use this term in the context of the rheological behaviour of sediment, where rheotropic states range from completely fluid to solid (“strongly cohesive”, p.36). Structural responses range from flows where there is a “complete loss of cohesion” (p.37) to fractures formed by brittle failure.
A. Maltman’s (1984) essay was one of the first to look critically at what constitutes SSD and its distinction from HRD. A couple of his comments reflect the vagueness of the term. For example, if the structures can be related directly to sedimentary processes, then the term penecontemporaneous deformation might be more appropriate (as is the case for convolutions in turbidites). He also suggests the term pre-lithification instead of soft, but this is no less vague a term and could be problematic in weak rock (noted above) or carbonates where lithification can begin soon after deposition (such as beachrock and sea floor cements).
Maltman’s more specific recommendation is to look at grain textures at the microscopic scale. Deformation in soft sediment will generate grain rotation and translation without structural change to the grains themselves. In lithified rock the same processes are likely to produce deformation within grains – fractures, lattice dislocations (e.g., quartz), twin or cleavage plane displacement (e.g., feldspar), or pressure solution. The distinction between lithified and pre-lithified textures in the context of SSD is useful and has been used in more recent publications (e.g., Waldron and Gagnon, 2010; Alsop et al., 2017).
Conditions leading to soft sediment deformation
Saturation of sediment:
Sediments deposited in subaqueous conditions are completely saturated. Rapid deposition, as is the case for sediment gravity flows and some fluvial deposits, commonly leads to leads to excess interstitial water. Over-saturated sediment is inherently unstable; dewatering begins soon after, and in some cases during deposition.
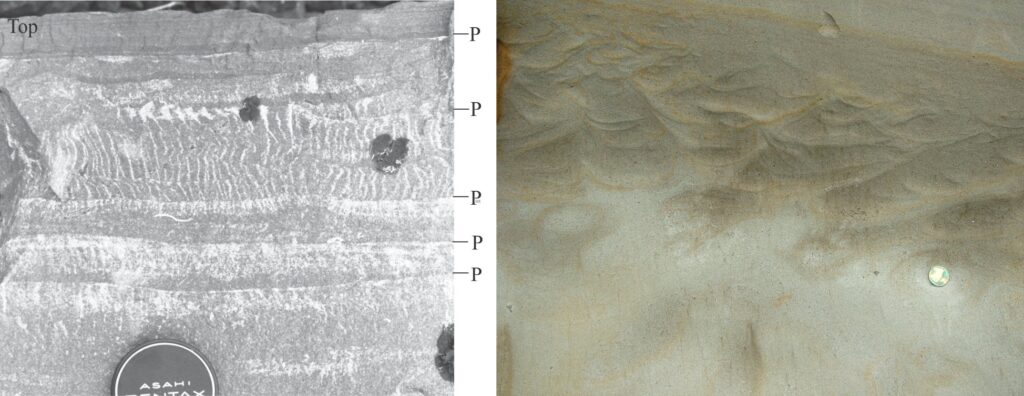
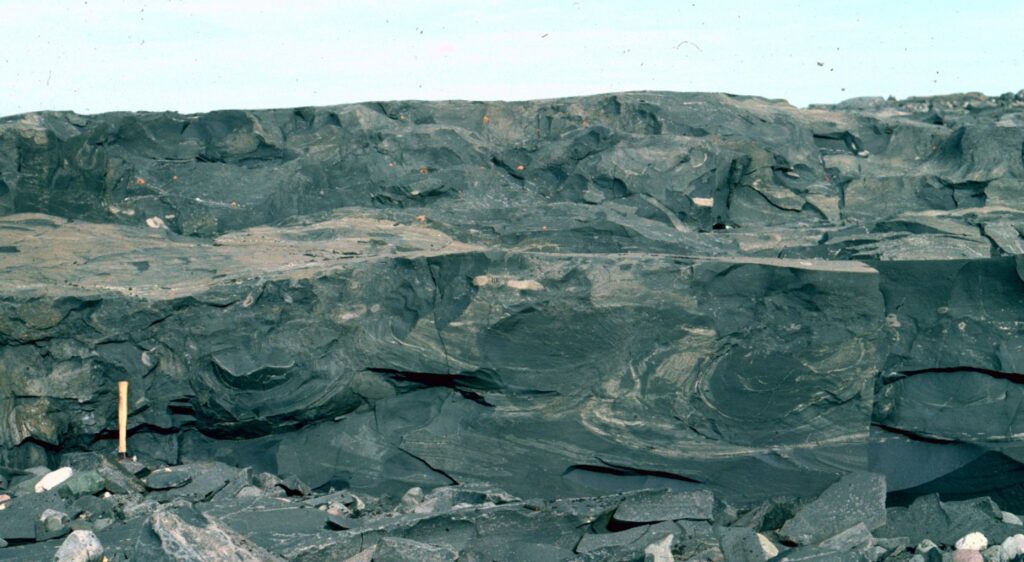
Compaction:
Compaction is a process that continues pretty much for the life of a sedimentary basin. The earliest stages of compaction are most important for SSD. Compaction begins immediately following deposition. It is primarily a gravity-driven process that rearranges grain fabrics, moving grains into more stable packing geometries. In the process, interstitial water is expelled. If sediment permeability is reasonably isotropic then water expulsion will be diffuse. However, most deposits contain textural and fabric discontinuities such as grain-size grading or crossbedding and will also inherit permeability gradients that either focus the flow of escaping water or impede its flow. Both conditions can generate overpressured fluids – deposits with compartments of overpressured fluid are inherently unstable because cohesive strength and shear strength tend towards zero.
Common triggering mechanisms:
- Differential compaction and dewatering in depositional units such as turbidites, and rapidly deposited fluvial deposits. This mechanism can also be generated during slumping, particularly in the core zones of slump folds.
- Excess sediment loads: Rapid accumulation of fluvial-derived sediment across shelf-slope breaks and pro delta slopes during storms can increase slope gradients beyond their stable angle of repose.
- Seismic shaking (earthquakes, volcanic eruptions): This mechanism is commonly invoked as a trigger for slope failure. Spectacular examples occur on the margins of the Dead Sea Basin (e.g., Alsop and Marco, 2011), and the Early Miocene Waitemata Basin (Sporli and Rowland, 2007; op cit). Subaqueous failure can be triggered across surfaces with gradients as low as 0.25o, including continental shelves and slopes, platforms, and delta plains. Seismically induced shaking can also trigger collapse of volcanic edifices (e.g., sector collapse), the head-wall regions of submarine canyons and shelf-edge gullies, and the tectonically steepened margins of accretionary prisms at convergent plate margins. An example of the latter is the Ruatoria debris avalanche located offshore East Cape, New Zealand along the Tonga-Kermadec-Hikurangi subduction margin (Collot et al., 2001).
- Seismic shaking is also responsible for liquefaction of saturated sediment. This has been reported on numerous occasions associated with earthquakes –examples commonly lead to building foundation failure, and expulsion of fine sediment via fluidized flow.
- Diapirism: Salt diapirism can drastically change bathymetric profiles across marine platforms and shelves. Rapid, buoyant intrusion of mud beneath delta slopes can change local slope gradients and even breach the sea floor.
- Storm waves and tsunamis: The passage of storm or tsunami waves can induce sudden increases in sea floor sediment pore pressures, rendering them prone to failure (Alsop and Marco, 2012). This has happened several times along the Mississippi delta front during cyclic loading by hurricane-generated waves (Maloney et al., 2020).
Some criteria for SSD recognition
The primary tasks we need to undertake to recognizing deformation as soft sediment in the rock record involve deciphering the timing, the physical state of the sediment, and the combinations of rheological behaviours (did it behave as a hydroplastic or fluid?). For any interpretation, criteria that include fluid behaviour expressed as structures developed during liquefaction or fluidization are probably the least ambiguous.
- Deformed sediment sandwiched between non-deformed strata. Deformation in this stratigraphic arrangement is usually interpreted as predating the overlying depositional event. The interpretation is strengthened where underlying and overlying strata have similar composition and lithofacies, a common feature of turbidite-flysch successions. Small scale examples of this are the convoluted laminae in turbidite beds that are overlain by non-deformed, fine-grained deposits from the same turbidity current flow unit. However, the character of upper and lower contacts to the deformed package are important.
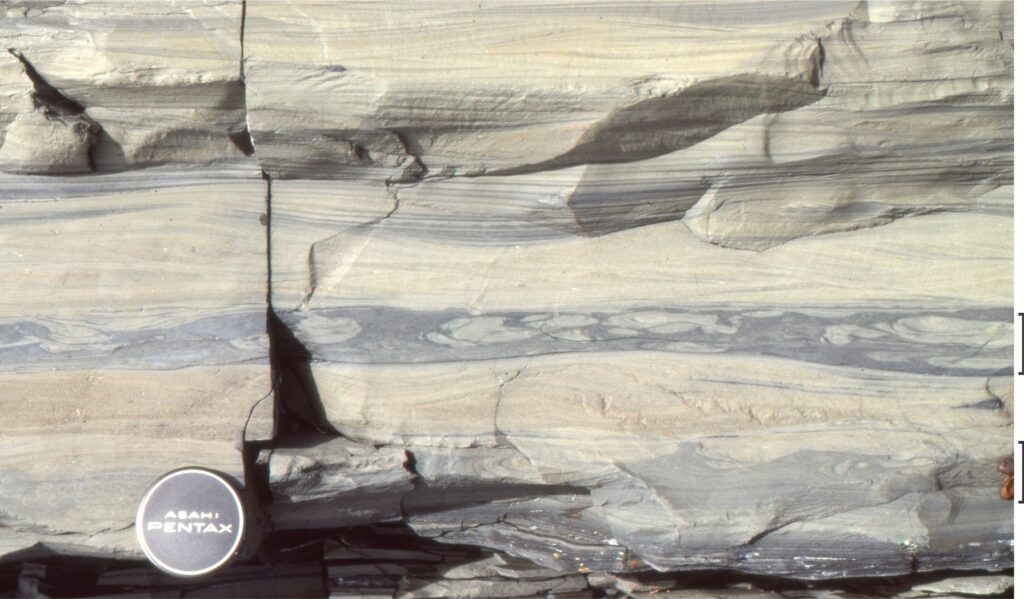
- Conformable upper contact – in this case overlying penecontemporaneous sediment will infill lows and drape highs over the deformed beds; the example from Ridge Basin (below) demonstrates this. The interpretation is ambiguous where the upper contact is an unconformity or structural discordance.
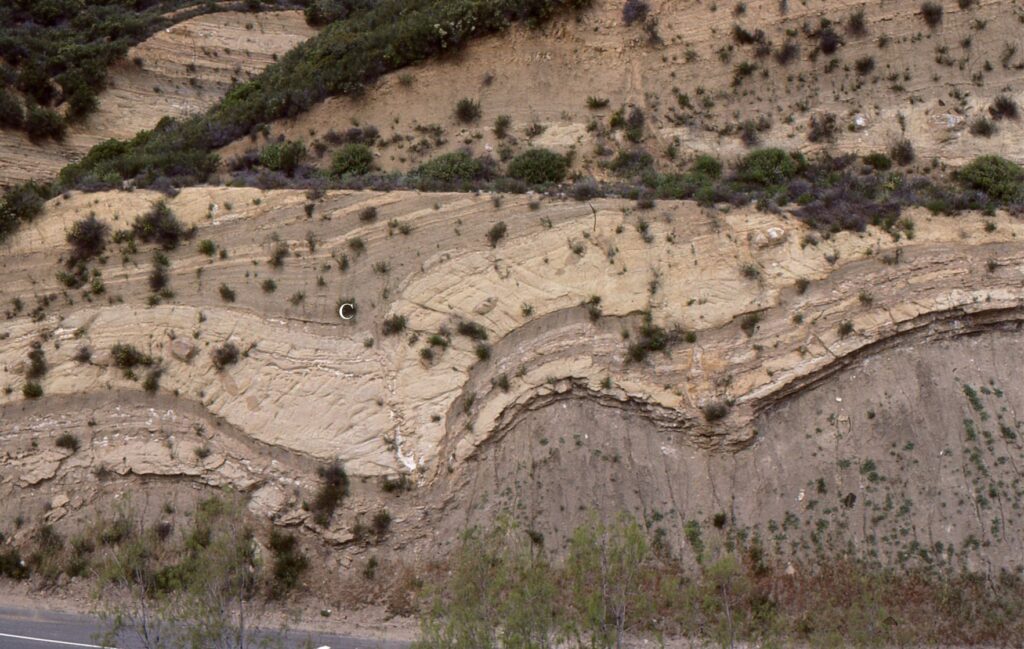
- Eroded upper contact – Truncation of folds and faults is useful if the overlying strata are the same lithofacies or lithofacies assemblage. The erosional discordance in this case can be reasonably inferred as short duration, rather than a fully-fledged unconformity.
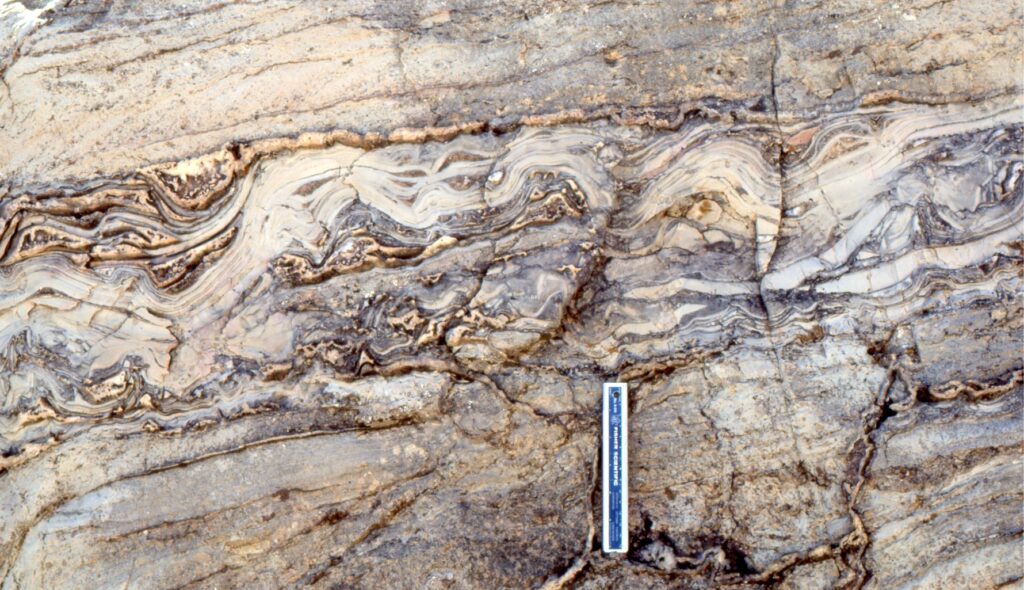
- Conformable basal contact – deformation occurred soon after deposition. Load casts are common examples at small scales.
- Basal glide planes (detachment surfaces) – these are structural discordances in both soft and hard rock deformation. They are common beneath large slump and slide packages. The presence of any deformation such as drag folds or brecciation below the detachment is ambiguous – this can occur in both soft and hard rock domains. However, if dewatering structures develop beneath the detachment (because of increased pore pressures from the imposed load) then this indicates a degree of water saturation consistent with SSD, particularly if any of these structures pass through the glide plane into the overlying deformed package.
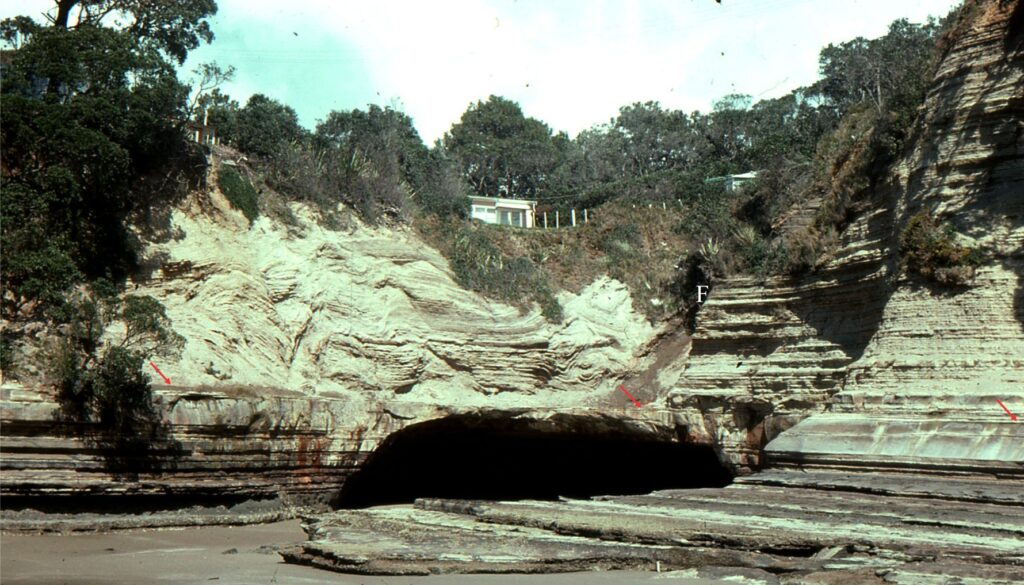
- Large-scale slump packages (also called Mass Transport Deposits – MTDs) tend to be structurally incoherent. The orientation of individual folds and faults is commonly variable. Slump folds can detach and rotate relative to other structures in the MTD. Contrast this style with regional tectonic deformation that tends to produce much greater degrees of coherence among folds (at all scales – as in reasonably consistent S-Z fold vergences), faults, and penetrative structures such as axial planar cleavage.
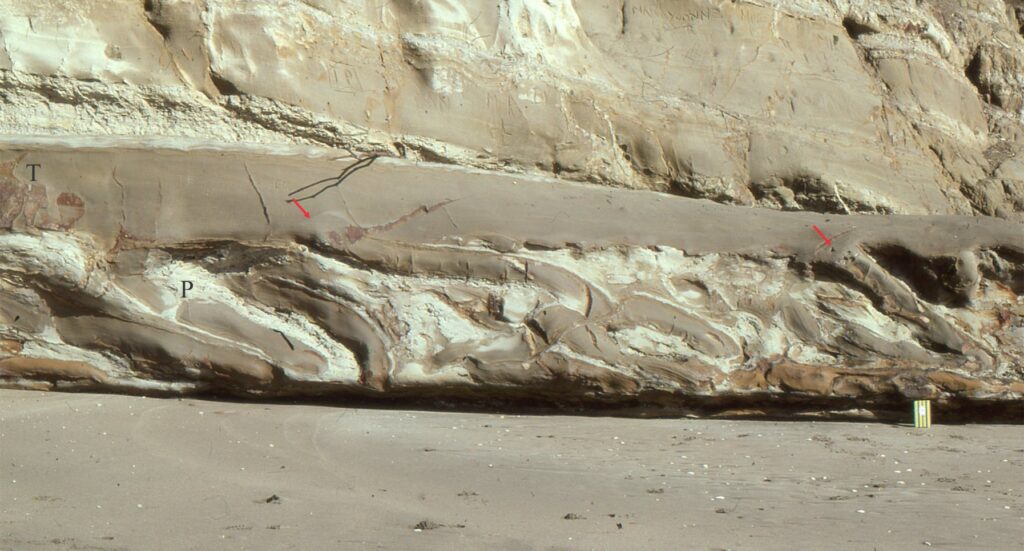
- Incorporation of different rheological behaviours in the same deformed package. Slumped strata commonly contain structures formed by hydroplastic flow, brittle failure, and liquefaction. The first two types are also characteristic of hard and weak rock deformation, but the presence of structures resulting from liquefaction provides a convincing argument for SSD.
References not linked in the text
J.R.L. Allen, 1982. Sedimentary Structures Their Character and Physical Basis Volume II &, Physical Sedimentology SSD Chapters 8, 9. Focus on liquefaction and fluidization.
J.R.L. Allen, 1985. Principles of Sedimentology. Chapman & Hall, London. 272 p.
C.E.B. Conybeare and K.A.W. Crook, 1968. Manual of Sedimentary Structures. Commonwealth of Australia, BMR Geology and Geophysics. Bulletin 102. 327 p.
F.J. Pettijohn, 1949. Sedimentary Rocks. Harper & Brother, New York. 526 p.
H.-E. Reineck and I.B. Singh. 1975. Depositional Sedimentary Environments. Springer-Verlag, Berlin, 439 P.W.H. Twenhofel. 1950. Principles of Sedimentation. 2nd. Edition. McGraw-Hill, 673 p.