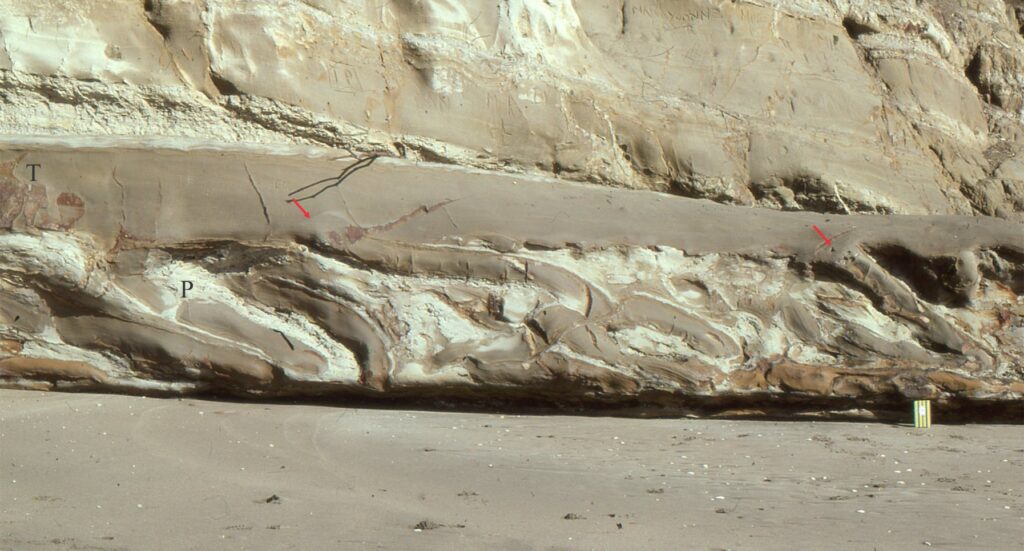
The rheology of soft sediment and weak rock. This post is part of the Lithofacies Series.
See the companion post – Soft sediment deformation
Rheology is defined as the “science of deformation and flow of matter” (Society of Rheology). The word itself derives from the Greek rheo, meaning flow. Although the mechanics of flow have been studied for centuries, the term was coined in 1920 by Eugene Bingham. Most rheological studies are concerned with materials that exhibit viscous and plastic behaviour. The study of rheology has its origins in basic mechanics.
Rock-fluid mechanics
Rock mechanics generally deals with three basic models:
- Elastic behaviour: This applies to solid materials that deform under applied stress and return to their original state when the stress is removed. There is no permanent deformation unless the elastic limit is exceeded, at which point the material will deform permanently, either by brittle failure or ductile flow, depending on variables such as temperature, confining pressure, and strain rate (i.e., deformation rate). Most materials exhibit some degree of elasticity.
- Viscous behaviour: Viscosity is the inherent resistance to deformation in a fluid. It is an intrinsic, rate-dependent property of any material that acts as a fluid (including solids). Dynamic Viscosity is defined as the proportionality constant in the function that maps shear stress and strain rate; it is calculated from the slope of a stress-strain curve.
The terms liquid and fluid are sometimes considered synonymous – they are not. All liquids (and gases) are fluids, but the converse does not apply. Think of the difference this way – a liquid poured into a container will instantly assume the shape of the container; it does not have the strength to resist this shape change. A fluid on the other hand will deform continuously – in the case of a liquid like oil or water, the deformation is extremely rapid; a lump of bitumen will also deform continuously but slowly. The difference between these rates of deformation is fundamentally a product of material viscosity.
- Plastic behaviour: This category refers to materials that deform permanently beyond their elastic limit (excluding brittle failure). Failure occurs at atomic and microscopic scales – mechanisms that have been identified include breaking and reforming of atomic bonds, dislocations in crystal lattices, slip along twin or cleavage planes, and microfractures in rock matrix. There are several models that describe different plastic behaviours; the more common (and simplest) models applicable to sedimentary processes are shown below.
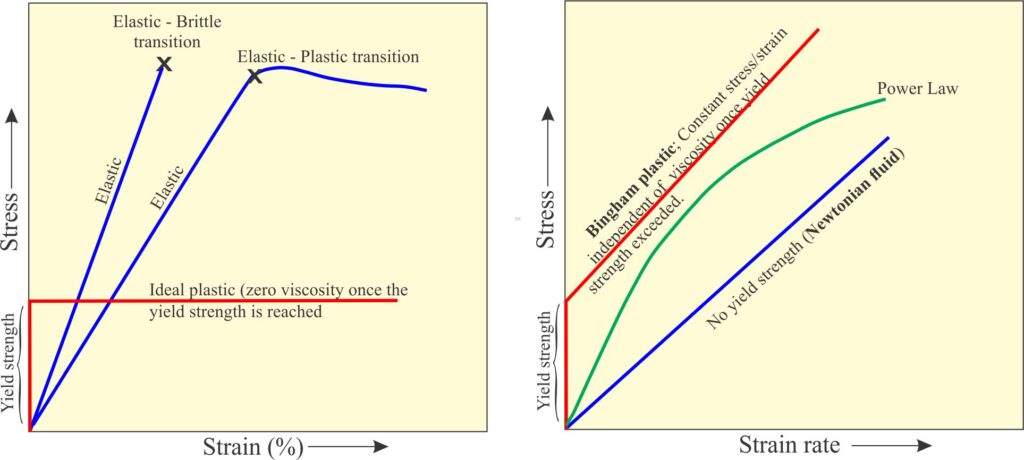
Right: Stress-strain rate curves are used to illustrate the time-dependence of material deformation according to each rheological model. For Newtonian behaviour, stress is proportional to the rate of deformation and viscosity is constant (viscosity is the slope of the straight-line graph). In the Bingham model, applicable to some mud flows, viscosity is also constant once the yield stress is reached. However, not all models have linear relationships between the shear stress and the shear rate – the example shown here the Power Law model where viscosity is not constant; there is no yield strength in Power Law behaviour. Graph plots from various sources including Middleton and Wilcock (1994)
Engineering applications typically deal with liquids or gases but are commonly extended to solids that flow in response to applied stress. In this context, the reference to fluids is appropriate because a fluid is defined as a material that deforms continuously when a shear stress is applied. Solids in comparison can resist shear stresses up to the point where forces exceed the material strength; in other words, deformation is not continuous.
Many industrial processes rely on specific rheological behaviours of materials, for example mined mineral separation (flotation), borehole drilling fluids (for drill-bit lubrication, suspension of drill cuttings, and stabilization of borehole walls), produced fluids such as oil and gas or geothermal fluids, fluidization processes, paint (that requires sufficient cohesive strength to counter gravity-imposed shear stress), and processes that involve gelling (as in food production).
Geologists apply concepts of rheology to explanations of phenomena such as hard and soft rock deformation, and numerous forms of fluid flow. We also apply rheological concepts to physical experiments and models. In addition to the standard solid, liquid, and gas states, we also consider complex mixtures:
- Liquid states, commonly manifested as flowing surface or subsurface water, or lava
- Solid states, as in crustal hard rock deformation, or characterization of deep Earth flow of mantle rock.
- Liquid-solid mixtures (e.g., sediment gravity flows, liquefaction, magma-crystal mush).
- Gas-solid mixtures (e.g., pyroclastic density currents),
- Gas-liquid mixtures like those envisaged for hydrocarbon migration and production.
Fundamental rheological behaviours
Rheology is the study of how materials deform with respect to the rate of applied shear forces, time (measured as the duration that forces are applied), and space (for example a change in shape). Material behaviour is also dependent on temperature and pressure. As noted above, different rheological behaviours can be expressed graphically in terms of stress – strain rate, or stress-strain curves. There are two fundamental types of behaviour:
- Newtonian behaviour: Newtonian fluids deform immediately when stress is applied such that there is a linear relationship between stress and strain rate (i.e., as stress increases, so too does strain rate if temperature and pressure are constant). The slope of the straight-line plot is the dynamic viscosity. The straight line passes through the origin. Newtonian fluids have no inherent strength. The most common natural example is water, but many hydrocarbons and some highly fluid lavas also obey these conditions.
- Non-Newtonian behaviour: Most natural materials, including some fluids do not deform the instant stress is applied – they begin to deform only when stress levels exceed their natural strength (the dimensions of stress and material strength are the same – M L-1 T-2; the SI unit is the Pascal). The stress value at which brittle deformation begins is called the elastic-brittle transition, and the beginning of ductile-plastic deformation the elastic-plastic transition. Non-Newtonian rheology covers a range of material behaviours and deformation mechanisms.
Most natural sedimentary materials exhibit some kind of plastic behaviour when stress is applied. The simplest behaviours are described by linear stress-strain or stress-strain rate relationships where viscosity is constant once the yield stress is reached (e.g., ideal plastic, Bingham plastic). However, the rheological behaviours of most materials, particularly during soft sediment deformation, are more complex combinations of elastic-plastic-fluid properties where viscosity, strain rates, and applied stresses can vary from one part of a deforming slump package to another.
For example, consider the kinds of mechanical transitions that take place during formation of a slump fold. An initially coherent sedimentary package begins to move downslope, deforming beyond its elastic limit, becoming increasingly fluid (decreasing viscosity). Plastic deformation results in folding. If fluid pressures increase locally because of loading or compression in fold hinges, then sediment can become liquefied at which stage it loses much of its shear strength and begins to behave more like a Newtonian fluid. When downslope movement ceases, dewatering increases viscosity where behaviour passes through a later plastic stage to a relatively consolidate, stable deposit. All these transitions can occur at different times and in different locations within a single slump package.
Rheological behaviour relevant to SSD
Soft sediment deformation is a catchall term for penecontemporaneous deformation of soft sediment and weak rock. These materials commonly behave in the following ways – note that all three rheological behaviours can act in tandem in a single deformation succession depending on lithology, the distribution of permeability pathways and barriers, viscosity, and strain rate (refer also to the outcrop examples shown below):
- Elastic solids: Most geological materials have some degree of elasticity. These materials can transition from elastic to brittle or elastic to plastic behaviour depending on factors such as material strength , strain rate, temperature, and confining pressure (see the stress-strain graphs above). Brittle failure is possible in soft sediment if the strain rate is high, which it invariably is during slump and mass transport events (MTDs), or if sedimentary layers are partly or fully lithified (e.g., some vadose zone and sea floor carbonates). It is commonly manifested as fractures, pull-apart structures, and boudinage in relatively coherent beds. In contrast, most non-recoverable soft sediment folding or distortion is plastic.
- Viscous fluids: J.R.L. Allen (1982) notes that liquefaction of sandy sediment is inevitable during soft sediment deformation. Liquefied sediment-water mixtures have negligible shear strength and high viscosities. Flow is driven by a combination of elevated fluid pressure and gravity. Fluid pressures can be elevated by simple loading, or confinement in fold hinges. Structures formed by flow include intricate, disharmonic folds, and thickening or thinning of sedimentary layers. Subsequent dewatering re-establishes more stable grain-to-grain packing, and in the process can produce dewatering pipes and channels that carry fine sediment to the surface.
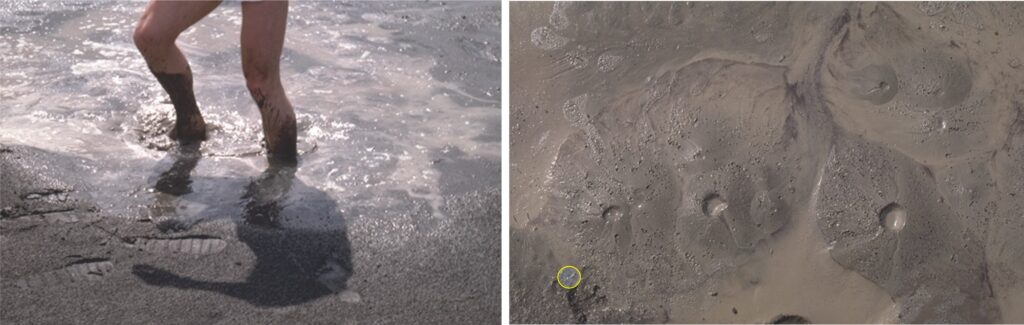
- Plastic or hydroplastic deformation (the term hydroplastic is commonly used for deformation of soft sediment). SSD folding is a hallmark of hydroplastic behaviour. Fold amplitudes range from a few millimetres to many 10s of metres. Fold geometry is determined in part by the degree of consolidation or lithification, and bed thickness (thick beds commonly form larger, more open folds) – not unlike hard rock deformation. Carbonate deposits that undergo early lithification may be prone to fracturing and faulting around fold hinges (illustrated in two examples below). In less consolidated deposits, ductile flow may thicken beds in hinge zones, with concomitant bed thinning along fold limbs.
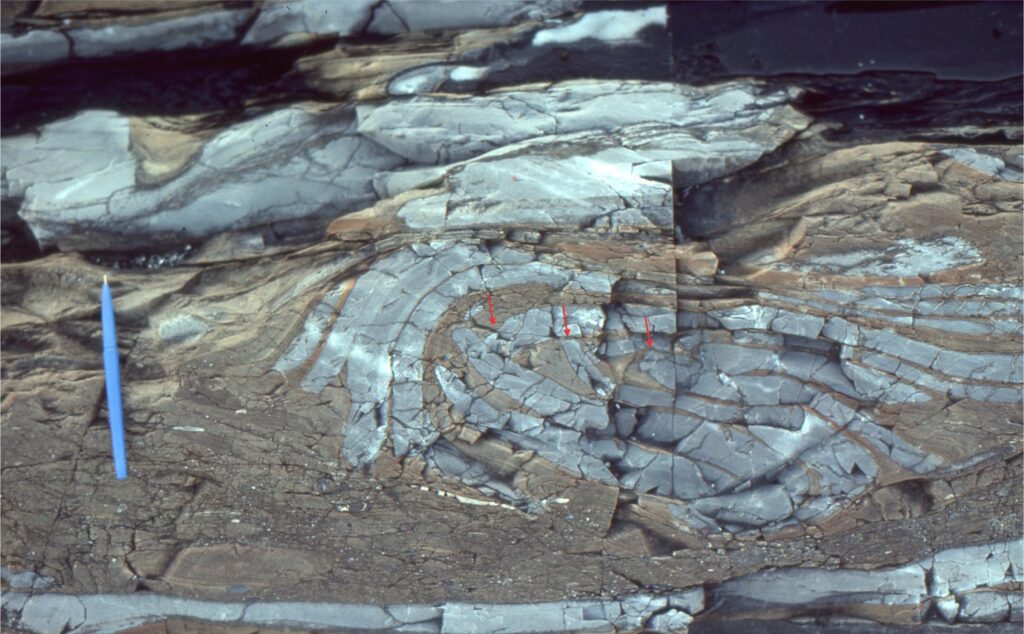
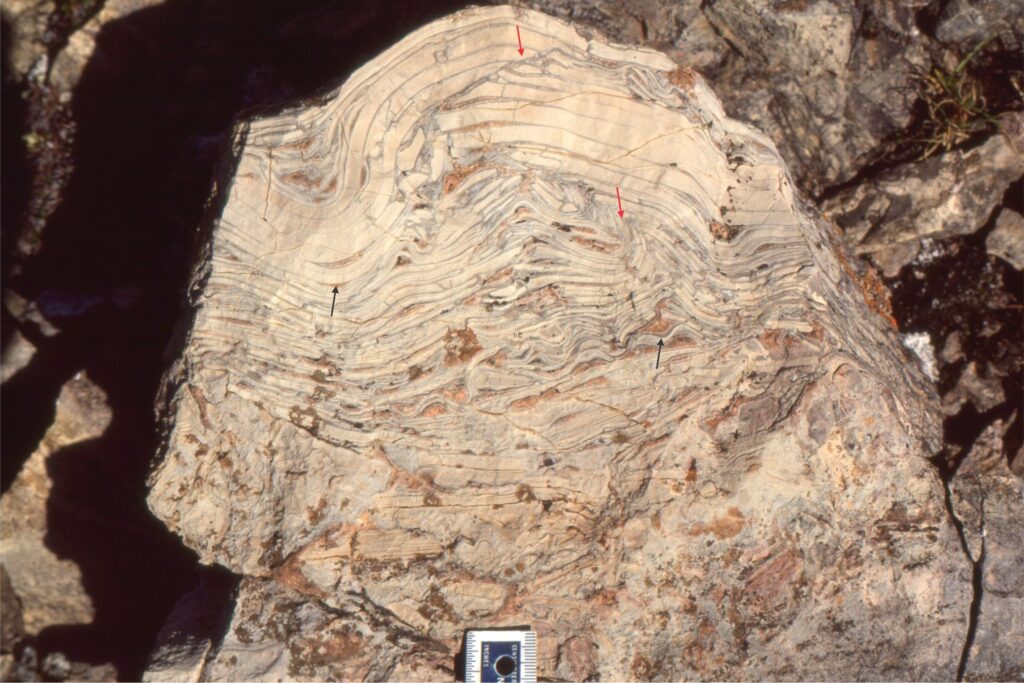
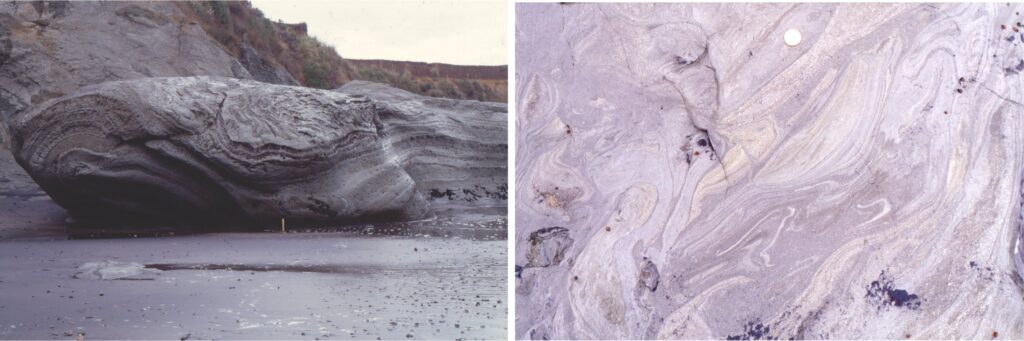
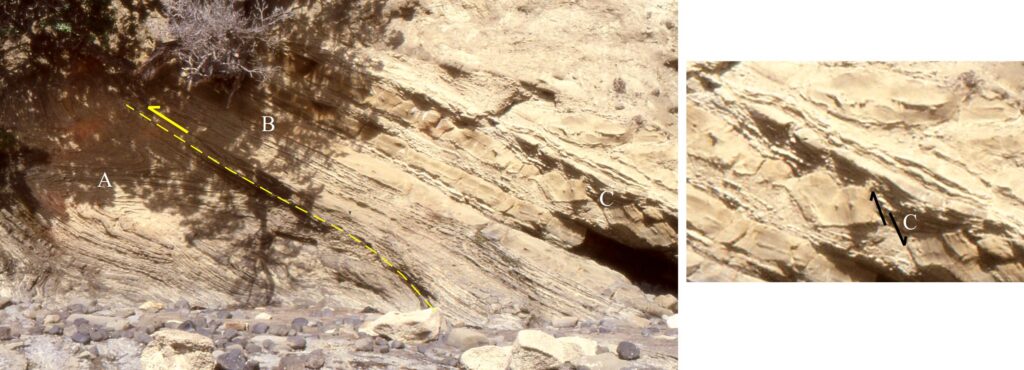
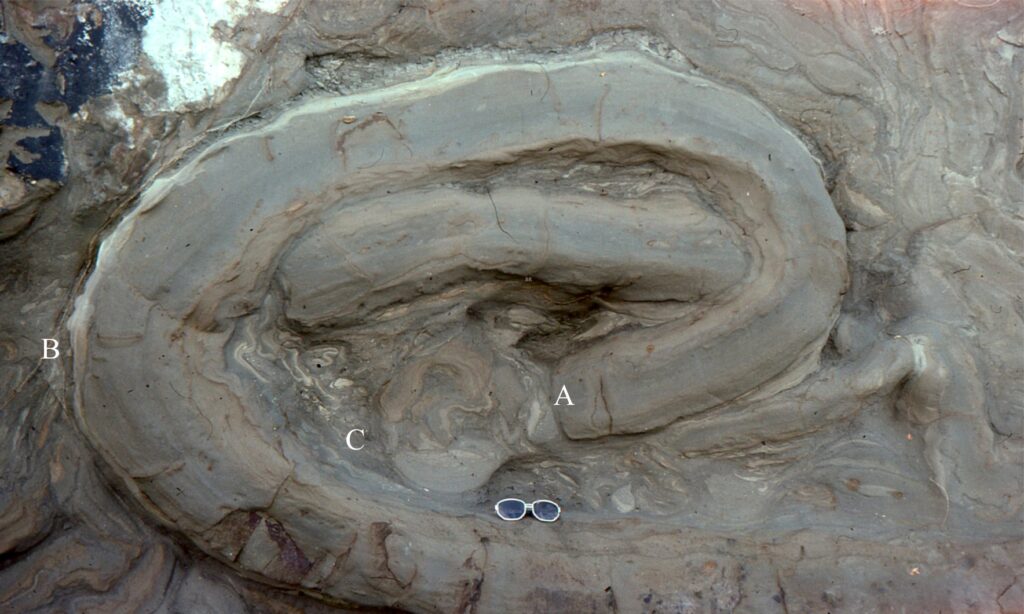
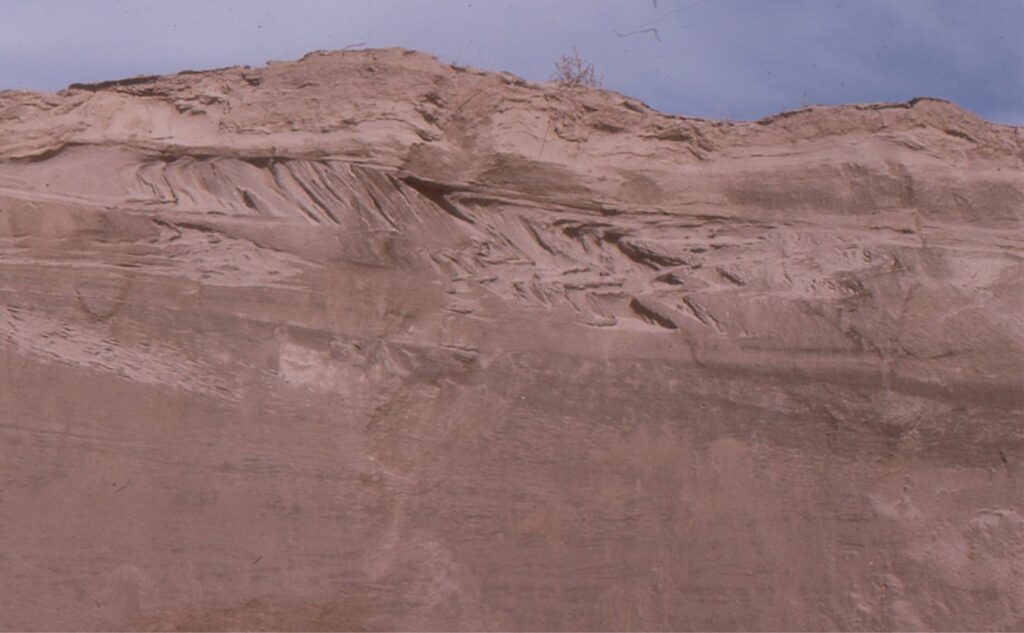