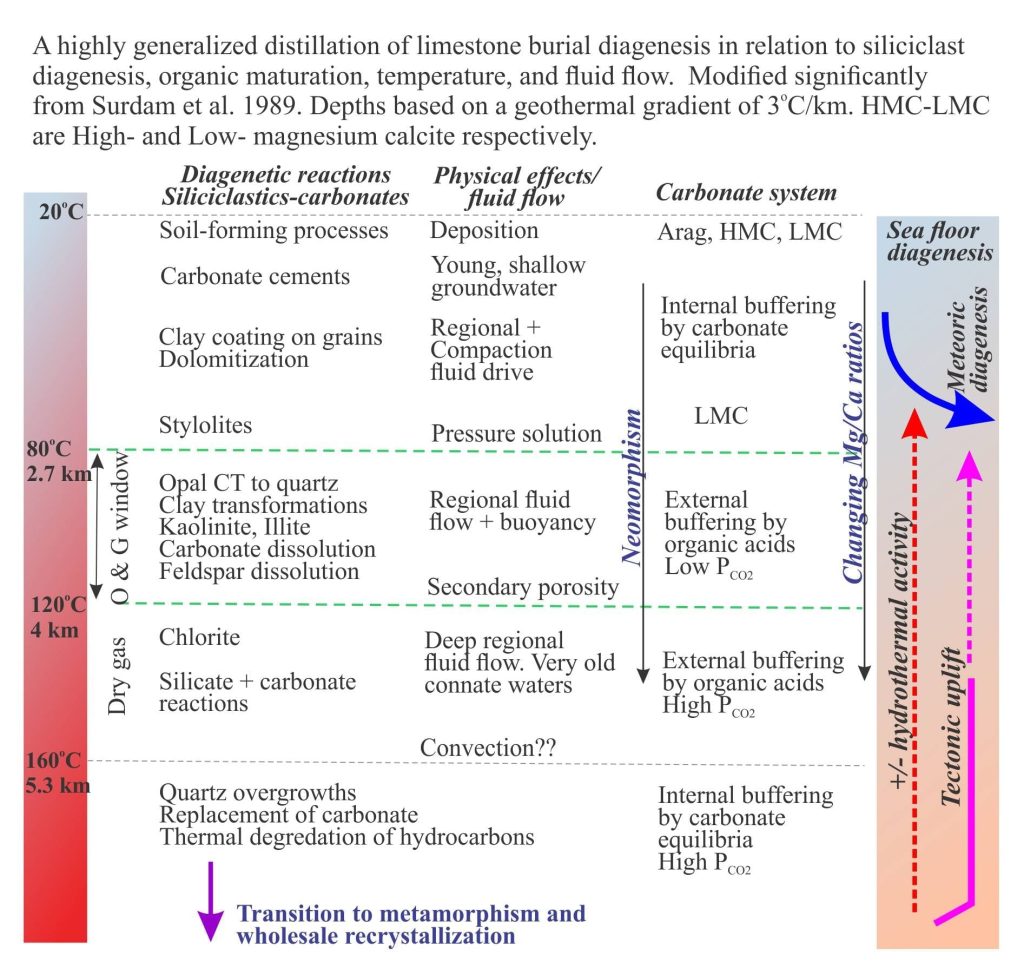
This is part of the How To…series on carbonate rocks
Some general statements about the diagenesis of limestones during burial.
As sediment is buried, the combined effects of temperature, pressure and changing fluid composition act to lithify, overprint, and even obliterate all previous diagenetic histories. In limestones, the original sediment components and any early cements formed during seafloor or shallow meteoric diagenesis, are cemented, replaced by more stable carbonate phases, or recrystallized. Burial diagenesis is governed primarily by:
- Increasing temperature as a function of the local geothermal gradients,
- Increasing hydrostatic pressures with depth and sediment/water loads,
- Reactions resulting from organic maturation where pH buffering and changes in pCO2 drastically shift the stability of carbonates,
- Reactions involving silicates (especially clays) that also change fluid composition and pCO2,
- Tectonically induced faulting and folding that can alter permeability pathways, and
- Tectonic uplift that reduces ambient temperatures and pressures and exposes indurated rock to meteoric conditions.
Temperature becomes important at relatively early stages of burial – keep in mind that under average geothermal gradients the temperature increases 3oC for every 100m depth. Thus, even at depths of only a few 100m, rates of reactions can increase several fold and new reactions may come into play, particularly those involving organic maturation (decarboxylation).
With increased depth and residence time, fluid compositions that were originally close to that of seawater, now include many more dissolved aqueous components, perhaps with increased salinity. The basic premise that groundwater is constantly flowing and that these waters are continually involved in chemical reactions, cannot be overstated. This also means that diagenetic reactions at one location may be caused by fluid compositions generated elsewhere in the sedimentary basin.
The important diagenetic processes that take place during sediment burial are described below; most apply to siliciclastic deposits as well as carbonates. Some of these processes will be treated in greater detail in separate articles:
Compaction
All sediments undergo compaction. Compaction begins soon after deposition as the load from overlying sediment increases. Two things happen in the first few 100m of burial:
- grains move closer together, reducing the effective porosity, and
- Interstitial water is driven off, guided in its transfer to other parts of the sedimentary basin by porous and permeable aquifers. These fluids participate in chemical reactions.
Compaction of mudrocks is much greater than that in arenites. Fluids derived from mudrocks will tend to have a greater diversity of dissolved cations and anions that will play a pivotal role in the diagenesis of coarser grained lithologies.
Pressure solution – Stylolites
This phenomenon has received a lot of attention that has generated several competing hypotheses (this is given greater prominence in a separate article). The central question is “how does pressure at grain-to-grain contacts result in mineral dissolution?”. We can reframe this question in the form of two conditions that must be met for the process to occur:
- Fluids at grain-to-grain contacts need to be undersaturated with respect to the mineral phase, most commonly calcite, (pressure solution is also found in siliciclastic rocks). The degree of saturation at these contacts may be quite different to that of the bulk interstitial fluids.
- Dissolved mass must be transferred from the site of dissolution. This requires sufficient effective porosity and permeability pathways.
Pressure solution can be widespread during early to intermediate stages of burial. In carbonate rocks it is commonly manifested as stylolites, saw-toothed shaped boundaries or planes that cut primary depositional fabrics such as bedforms, rigid biogenic structures like corals and stromatoporids, and stromatolites. The volume of ‘dissolved’ rock can be significant, resulting in a loss of stratigraphic thickness, and importantly for diagenesis, the transfer of large volumes of dissolved carbonate that participates in calcite precipitation.
Dolomitization
Dolomite is a common diagenetic product of deeply buried carbonates, either as primary cement or replacement of calcite and aragonite. Dolomitization may be local or extend through entire limestone platform successions. Many Precambrian carbonate platform successions are completely dolomitized. Dolomite replacement can be fabric-selective where it faithfully replaces and mimics calcite and aragonite cements (e.g. fibrous and isopachous cements) and bioclasts, or it can obliterate these earlier fabrics. Dolomite may occur as scattered euhedral crystals in a limestone host, or completely replace the limestone.
Dolomite precipitation is another conundrum that geologists have grappled with for decades (the vast literature on the topic attests to this). Seawater is generally supersaturated with respect to dolomite and yet it is always a minor constituent of surface deposits, even in hypersaline environments. It is also difficult to precipitate experimentally under conditions at surface temperatures and pressures. When it does form it is nearly always disordered. Thus, dolomite precipitation at the surface is thermodynamically favoured but, it seems, is kinetically inhibited from forming by some combination of factors like insufficient Mg2+ concentrations, low Mg/Ca ratios, dehydration reactions of Mg2+ and Ca2+ at the crystal surface, or inhibition by dissolved sulphate (there is some experimental evidence for this).
Formation of ordered dolomite is clearly favoured during burial. In part this is due to increased temperatures that will increase reaction rates, changing Mg/Ca ratios, and the longevity of burial.
From a mass balance perspective, the concentration of Mg2+ is a problem, particularly where entire platform successions have been dolomitized. The amount of Mg available in HMC is insufficient. It is likely that Mg released from clay transformations in siliciclastic rocks is important. However, this requires efficient regional fluid flow to transfer the Mg to the carbonate platform.
Recrystallization and Neomorphism
Recrystallization is the general term for changes in crystal structure, commonly seen as an increase in crystal size. Here, many smaller crystals will recrystallize to fewer larger crystals. In carbonate rocks, recrystallization commonly involves no or little change in mineralogy; calcite recrystallized to calcite, dolomite to dolomite. This is called neomorphism. Neomorphism tends to destroy earlier textures and fabrics.
Neomorphism in buried carbonates is a chemical reaction. It will only take place if there is a thermodynamic advantage to do so. A good example to consider is the neomorphism of lime mud and micrite to coarser spar. Because of their size, mud-micrite crystals have very high surface areas. As burial temperatures and pressures increase so too does the degree of instability. The result is an increase in crystal size although the bulk composition does not change.
The oil and gas window
Bacterial mediated breakdown of organic matter begins soon after deposition. Temperature dependent maturation begins at about 60oC (on average about 2km depth) but is relatively slow until 80oC – the lower temperature limit of the oil-generation window where wholesale maturation begins. In addition to the production of hydrocarbons, hydrolysis reactions form a variety of organic acids. These acids may react further and release CO2 – these reactions are referred to as decarboxylation. Their importance for carbonate diagenesis lies in their ability to buffer pH and dissolve calcite.
Maturation of organic matter usually takes place in organic-rich mudrocks and coals. These are the hydrocarbon kitchens.
End note
Deciphering the relative contributions to burial diagenesis of all these processes (and others not mentioned such as hydrothermal alteration) requires all the tools at a petrologist’s disposal: optical microscope and Scanning Electron Microscope, X-Ray diffraction and X-Ray fluorescence, fluid inclusions, cathodoluminescence, stable isotopes, trace elements, geochemistry and thermodynamics, wireline logs, geopressures and fluid flow. All this data must be superimposed on a coherent stratigraphy and surface-subsurface maps. Then, perhaps, you may get an inkling of how carbonates respond to burial.
Links to other posts in this series:
Mineralogy of carbonates; skeletal grains
Mineralogy of carbonates; non-skeletal grains
Mineralogy of carbonates; lime mud
Mineralogy of carbonates; classification
Mineralogy of carbonates; carbonate factories
Mineralogy of carbonates; basic geochemistry
Mineralogy of carbonates; cements
Mineralogy of carbonates; sea floor diagenesis
Mineralogy of carbonates; Beachrock
Mineralogy of carbonates; deep sea diagenesis
Mineralogy of carbonates; meteoric hydrogeology
Mineralogy of carbonates; Karst
Mineralogy of carbonates; Neomorphism
Mineralogy of carbonates; Pressure solution
Useful texts: In addition to the texts and papers referenced in other posts in this series
H.G. Machel 2005. Investigations of Burial Diagenesis in Carbonate Hydrocarbon Reservoir Rocks. Available as a PDF
Art Saller, 2013. Diagenetic evolution of porosity in carbonates during burial. AAPG Powerpoint presentation available as a PDF.
C.H. Moore, 1989. Carbonate Diagenesis and Porosity. Elsevier, Development in Sedimentology, v. 46,