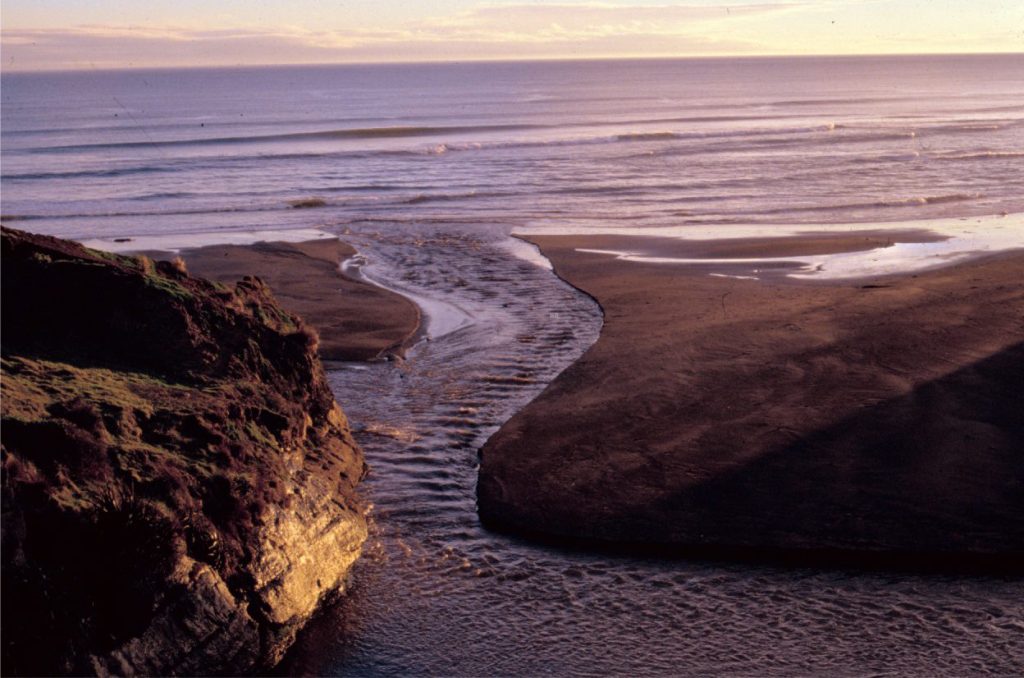
Use this link to read the introduction to the lithofacies series.
Here we look at the passage from antidunes to chutes and pools, and cyclic steps
Antidunes are usually the first bedforms that spring to our sedimentological consciousness when confronted by upper flow regime processes (UFR). We have a pretty good understanding of their formation and have developed criteria for recognising them in the sedimentary record – these criteria are not unequivocal, but they’re not bad!
The flow conditions that produce antidunes are part of a hydrodynamic spectrum where increasing velocities (and energies) give rise to a progression of flow conditions from chutes-and-pools to cyclic steps. We can observe these transitions in steep-gradient channels and river and alluvial fan channels in flood. At each stage of flow there is a sedimentary response. However, recognising these responses in the stratigraphic record is difficult, in part because we are not certain about what to look for. In addition, the wavelengths or crest separations of antidune, chute-and-pool, and cyclic step bedforms increase sympathetically with flow velocity which means their recognition in outcrop or reproduction in flumes becomes increasingly difficult. The relative size of each bedform is shown schematically in the diagram below.
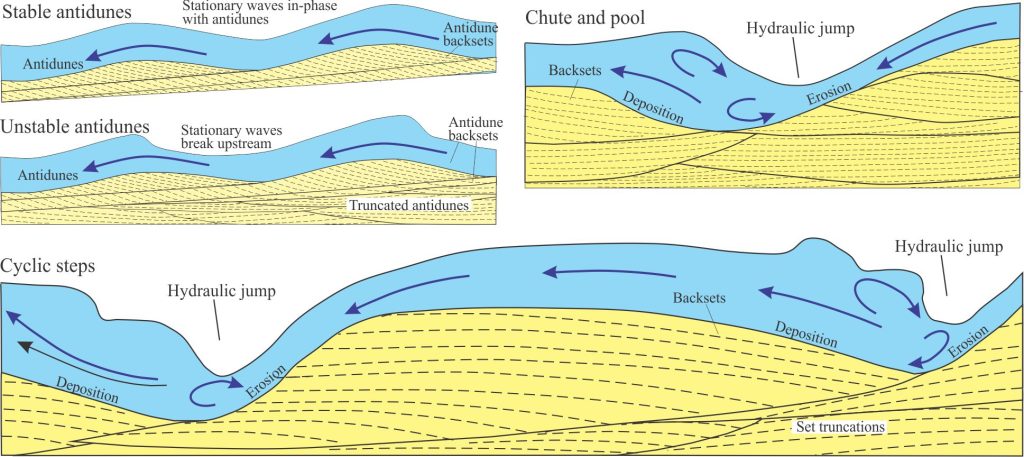
This post briefly describes the processes and possible depositional outcomes for UFR (supercritical) chute-and-pool and cyclic-step flow. But first we examine the hydrodynamic conditions that give rise to hydraulic jumps because they figure prominently in both chute-and-pool and cyclic step formation.
Hydraulic jumps
The transition from supercritical flow (Froude number Fr >1) to subcritical flow (Fr<1) results in an abrupt, instantaneous increase in flow turbulence and water depth. This transition is called a hydraulic jump. It takes place when the upstream propagation velocity of surface waves like those associated with antidunes (i.e., their upstream speed) equals the downstream-flow velocity. Hydraulic jumps may be stationary or migrate upstream or downstream depending on the relationship between upstream and downstream forces (Cartigny et al., 2014).
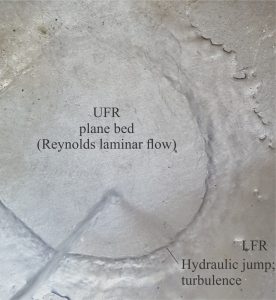
Energy is dissipated at a hydraulic jump, partly as flow turbulence but also to the substrate. The sand or gravel bed response is erosional scour where sediment is moved into suspension. The conditions immediately downstream of the hydraulic jump are subcritical (lower flow regime) such that sediment falls out of suspension and is deposited as backsets laminae.
Hydraulic jumps can occur as single events or as trains where several jumps mark the periodic transformation from supercritical flow upstream to subcritical flow downstream of the jump. The latter is typical of cyclic steps, but this kind of repetition can also occur in chutes and pools.
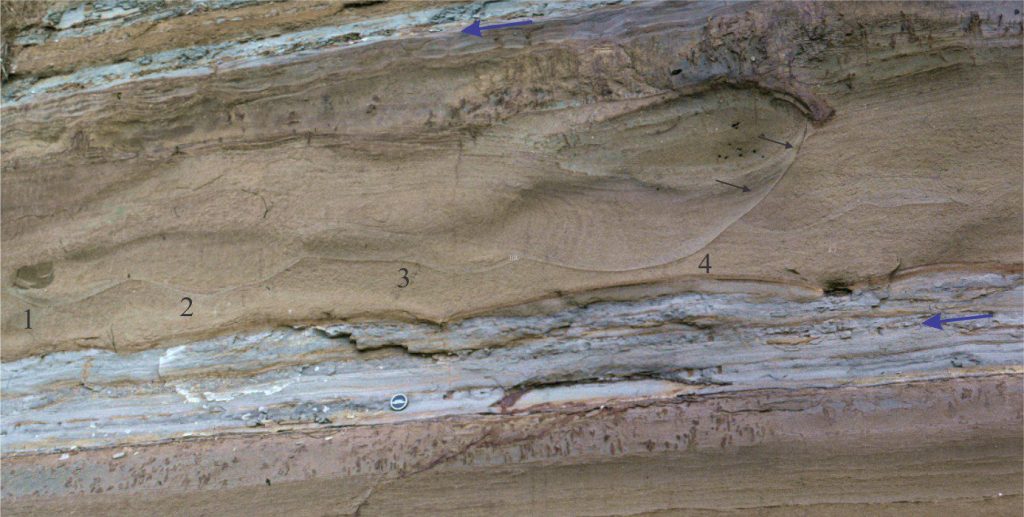
Chutes and pools
Chute and pool conditions usually develop at flow velocities higher than those responsible for unstable antidunes. Chute and pool morphology is centred on a hydraulic jump – upstream flow in the chute is supercritical, and immediately downstream flow is subcritical (the pool). Flows that gather pace downstream of the pool may produce a train of antidunes. Chutes and pools can also migrate upstream which means the hydraulic jump moves in tandem.
Flume experiments and numerical modelling have shown that deposition and preservation of chute-and-pool laminae depends strongly on the degree of aggradation during successive flow events. Deposition occurs primarily in the subcritical (tranquil) pool where backset laminae accumulate on the stoss side of flow. If aggradation is low, the passage of successive chutes and pools and antidunes, or the return to prolonged lower flow regime conditions, will probably leave little or no depositional record. If aggradation is high, then backset laminae can be preserved in overlapping lenticular sets. These backset lenses are like those produced by antidunes. However, distinction between antidune and chute-and-pool depositional architectures may be possible because:
- The wavelength between successive chutes and pools is significantly greater than that for antidunes which means the backset lenses should be larger, and
- The hydraulic jump may leave an identifiable scoured surface onlapped by backset laminae.
Cyclic steps
Increasing flow velocities under supercritical conditions give rise to a progression of surface wave forms, from stationary waves to chutes and pools, and eventually cyclic steps. The morphology of a cyclic step is basically that of a chute-and-pool, or a train of chutes and pools. Vellinga et al., (2017) provide a good summary of experimental data and numerical modelling of cyclic steps.
The ‘step’ part of a cyclic step refers to a relatively rapid decrease in flow velocity from supercritical to subcritical via a hydraulic jump. The hydraulic jump is erosive and causes the step to migrate upstream. The next stoss face, downstream of the hydraulic jump is defined as a zone where flow increases again from subcritical to supercritical.
Sedimentation occurs mainly on the stoss face. At its upstream extent, deposition by fallout from suspension (caused by the erosive power of the hydraulic jump) is followed farther downstream by bedload transport, until the next step forms. ’Cyclic’ in this context refers to the periodic fluctuations in flow that produce a train of steps.
The succession of wave forms and corresponding bedforms in increasing supercritical flow is accompanied by an increase in scale. Thus, the separation (wavelength) of wave crests and corresponding antidune, chute and pool, and cyclic step bedforms, also increases in sympathy with flow velocity (and Fr). Based on experimental and theoretical considerations, Alexander (2008) has suggested that the characteristic cyclic step wave-length is 100-500 times the flow depth.
This poses a potential problem for flume type experiments and the recognition of these supercritical bedforms in outcrops that typically lack continuity of exposure at this scale. High resolution imaging of the shallow subsurface, such as reflection seismic and ground penetrating radar, is a useful way to tackle this problem. Here are a few examples.
Large scale bedforms in some modern submarine canyons and slope channels are frequently attributed to deposition by supercritical sediment gravity flows. In an example from the South China Sea, 3D reflection seismic successfully imaged late Pleistocene and recent, 2D bedforms having amplitudes up to 32 m, and wavelengths from about 0.7 km to 6 km (Zhou et al., 2021).
Similar wave-like gravelly bedforms in Kaikoura Canyon (southeast New Zealand), at ocean depths exceeding 2 km, have amplitudes of 10-20 m but crest spacings of only 250 m (Mountjoy et al., 2018). Kaikoura Canyon, the sediment waves have arcuate (convex down-canyon) to straight crest lines and extend in a more or less continuous train for at least 30-35 km. Examples like these are commonly attributed to cyclic step deposition.
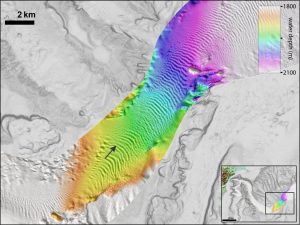
A combination of outcrop and ground penetrating radar (GPR) have successfully imaged supercritical bedforms in middle Pleistocene glaciogenic fan and Gilbert delta deposits in northern Germany Lang et al., (2017). GPR profiles image a series of scours along the Gilbert delta foresets, each scour filled by backset strata. Antidune bedforms occur downflow (and down dip) of the scours. According to Lang and his coworkers, the bedform transitions formed from surging, supercritical density currents.
G. Postma et al., (2021) use detailed sedimentological analysis of an Eocene deep water, delta-slope channel to lobe transition exposed in extensive road cuts to tackle this problem. They identified the up-slope migration of several hydraulic jumps (deep scoured surfaces) that were associated with backset and foreset bedding in chute-and-pool structures. Crest separations of the largest bedforms were 30-50 m.
Other posts in this series
Sedimentary lithofacies – An introduction
Ripple lithofacies: Ubiquitous bedforms
Ripple lithofacies influenced by tides
Tabular and trough crossbed lithofacies
Laminated sandstone lithofacies
Low-angle crossbedded sandstone
Hummocky and swaley cross-stratification