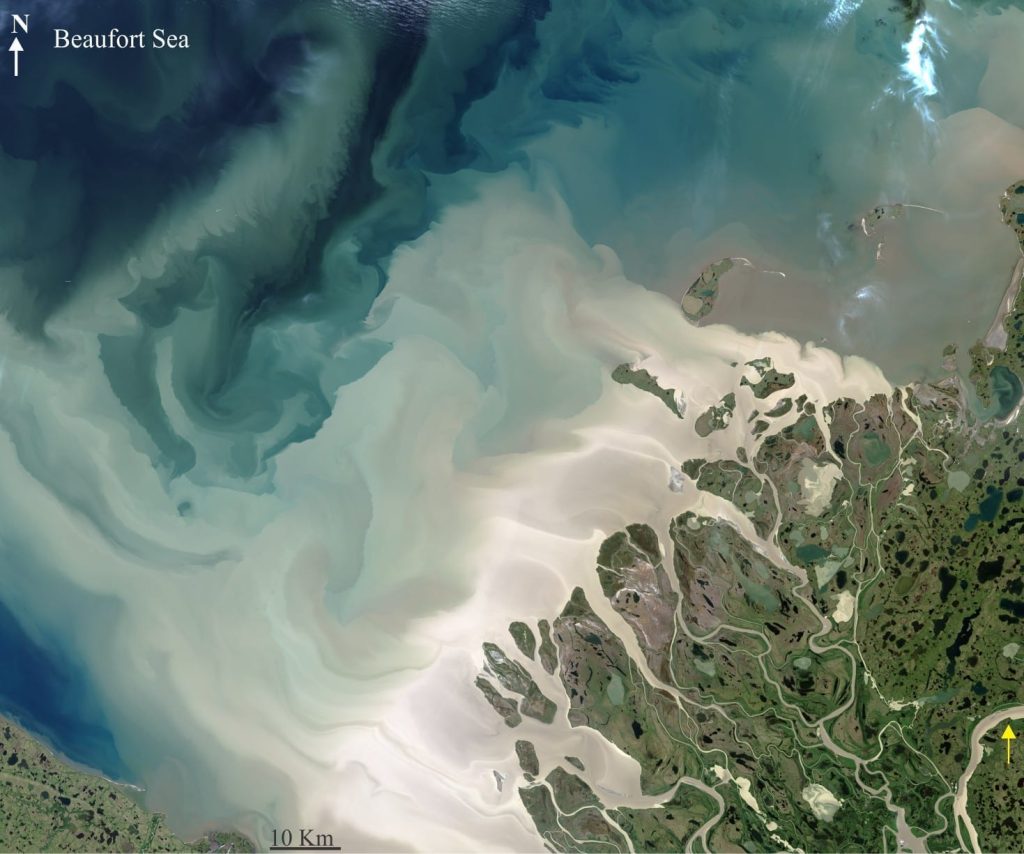
Image credit: NASA Earth Observatory, Jesse Allen https://earthobservatory.nasa.gov/images/90703/mackenzie-meets-beaufort
Depositional systems and systems tracts are central to the construction of sequence stratigraphic profiles.
Stand back and observe an active, modern delta, any delta – part of the Mackenzie delta is shown above (aerial and satellite images are best – any closer and you’ll get bogged down in detail). You will see an array of depositional environments: distributary channels, delta plains, crevasse splays, the front of the delta lapped by waves, and sediment plumes that will feed deposition on the prodelta slope. Some inactive segments of the delta may have been reworked into sand bars and spits that front a lagoon or embayment. All these environments are spatially distinct and yet they are dependent, one on the other. We can think of the entire delta as an integrated depositional system, where individual depositional environments are linked by all those physical, biological, and chemical processes that contribute to sediment supply and deposition, and the construction of a stratigraphic succession.
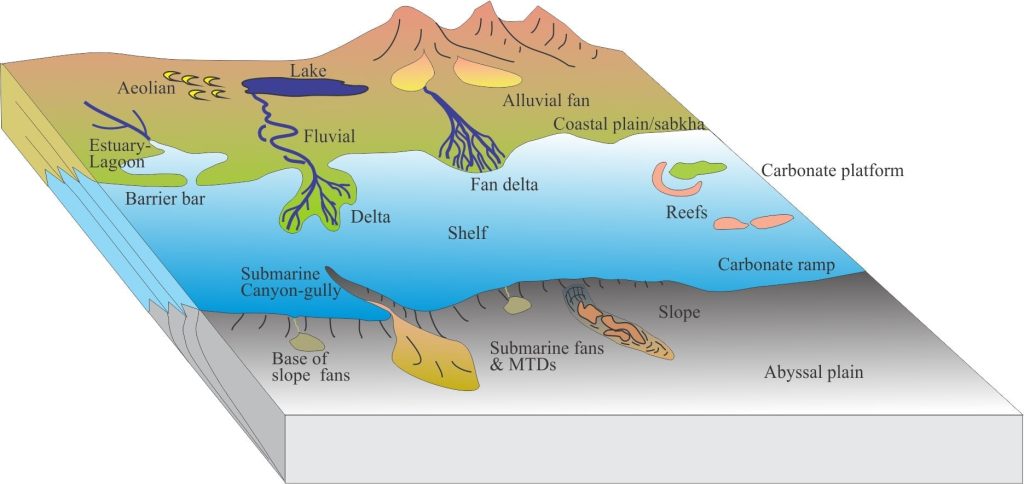
A modern depositional system can be described as a 3-dimensional array of genetically related environments. The expression “genetically related” is important. For the delta example, the distributary channels do not occur in isolation – sediment transport within the channels depends on fluvial and alluvial processes upstream, and a sink at the delta front terminus (i.e. the destination for sediment). Even though the processes may change from one environment to the other, they are inextricably linked.
We can also think of a depositional system in terms of its sedimentary facies and facies associations, for example a delta will include (among many other facies), crossbedded channel sands, peats and soils on the vegetated delta plain, interbedded sandstone-mudstone across the floodplain and crevasse splays, and mud-dominated facies in the prodelta. Applying the facies concept to depositional systems is crucial for interpreting ancient delta systems and unraveling the 4th dimension – time.
Thus, the definition of a depositional system, in the context of the rock record, can be expanded to include the 4-dimensional assemblage of genetically related facies. However, keep in mind that while we can observe directly the various processes and responses in modern systems, for ancient depositional systems we must infer the process-response of each sedimentary facies based on the kind of inductive reasoning permitted by uniformitarianism.
Some factors that control depositional systems, their facies, and stratigraphic architecture:
Both allogenic and autogenic processes will be important here.
- Topography of the depositional surface; for example, there are fundamental differences in sediment transport and deposition between the confinement of a channel and the relative openness of a tidal flat.
- The geomorphology of coasts, hinterlands, and ocean floors will have a strong influence on sediment routing, storage, and dispersal, and the corresponding depositional system. For example, the bathymetric expression of a continental shelf, slope, and deep basin will be reflected in the composition and geometry of their corresponding depositional systems.
- Sediment routing and dispersal by channelized flow, waves, tides, wind, volcanic eruption columns, or mass emplacement by slumps, slides and sediment gravity flows.
- Incident light through the water column (e.g. the photic zone) limits the availability of organisms that rely directly or indirectly on photosynthesis.
- Water chemistry: at its most basic, the differentiation of marine and non-marine; the state of carbonate equilibria or redox conditions.
- Time: sedimentary facies in succession imply the passage of time. Understanding the time component allows us to detect whether an alluvial fan depositional system is maintained or whether it changes into (for example) a fluvial system (such a change would have implications for other aspects of sedimentary basin evolution).
- Changes in baselevel will strongly influence the overall geometry of depositional systems, for example promoting retrogradation or progradation (also dependent on sediment supply).
- Tectonics and basin subsidence will have a strong influence on sediment composition, supply, and long-term fluctuations in sediment accommodation.
Systems tracts; Genetically linked depositional systems
In much the same way that specific environments are genetically linked within a depositional system, so too are different depositional systems linked. Thus, shelf and barrier island depositional systems are linked by virtue of the exchange of sand from one to the other, depending on their respective sediment budget requirements. Both of these depositional systems may be linked to deltas that are a major source of sediment. Likewise, sediment in coastal, delta, and shelf systems may be transferred to submarine fans depositional systems via submarine canyons and shelf-edge gullies.
Depositional systems that are contemporaneous and genetically linked comprise depositional systems tracts. The term was first coined by Brown and Fisher (1977) to aid interpretation of seismic profiles. It was subsequently commandeered by the Exxon sequence stratigraphers who expanded its meaning to represent a position on a sea level curve; hence the now more familiar terms highstand, lowstand, and transgressive systems tracts (e.g. Vail et al. (1977), Posamentier et al. (1988). Catuneanu (2006) provides an excellent historical summary of the various definitions wrought upon systems tracts over the intervening decades, particularly in the context of their timing relative to fluctuating sea levels.
Systems tracts consist of relatively comfortable stratigraphic successions. This requirement attempts to preclude the inclusion of major unconformities, such as subaerial unconformities that define the boundaries of stratigraphic sequences. However, most stratigraphic successions contain minor discordances and hiatal surfaces, common examples being shoreface ravinement surfaces, and omission surfaces. Thus, the qualification “relatively“ is used rather loosely.
Systems tracts do not adhere to any spatial scale or duration. The concept can be applied to 3rd order sequences or parasequences alike. Each systems tract is characterized by their stacking patterns; in high-order cycles this might be the stacking of lithofacies, in 3rd-order sequences the stacking of parasequences (keep in mind that stacking patterns are identified by their characteristic facies). The other determinants of a systems tract are its bounding surfaces.
It is generally accepted that genetic sequences can be described in terms of five systems tracts; for the Exxon-type depositional sequences there are the three noted above plus falling stage systems tracts (FSST) (Hunt and Tucker, 1992), and regressive systems tracts that form one half of Transgressive-Regressive (T-R) sequences (Embry, 2002).
Refer to the sea level curves and 2-dimensional (dip parallel) profile diagrams for each systems tract.
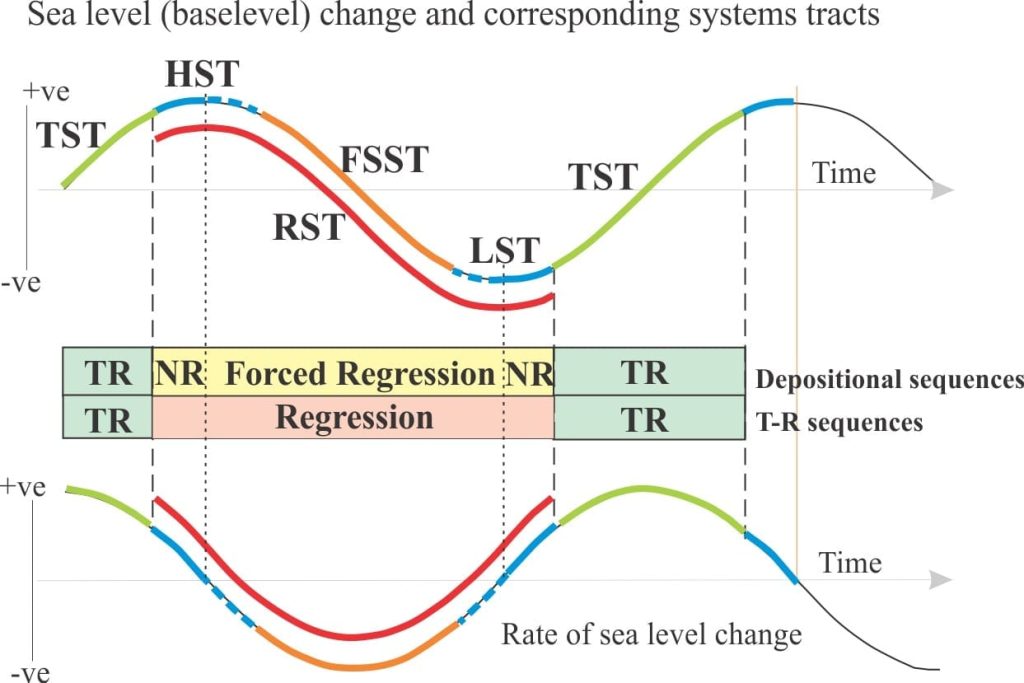
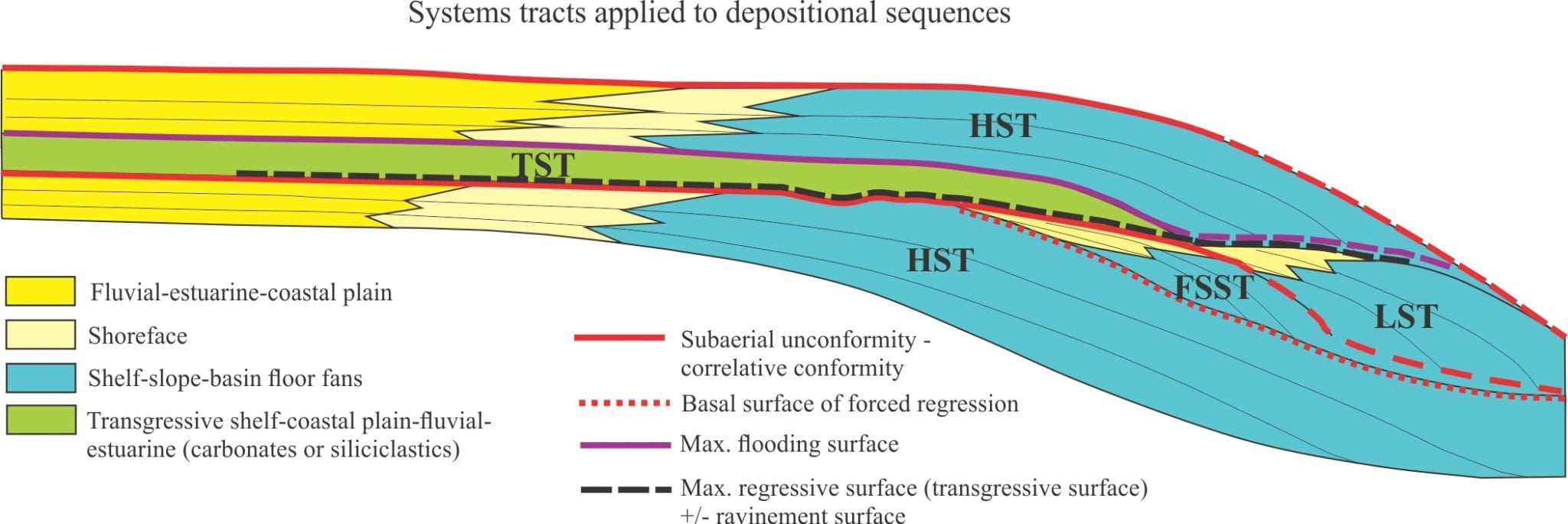
Highstand systems tracts (HST)
During the later stages of transgression, the rate of sea level (baselevel) rise decreases such that sedimentation rates exceed the creation of accommodation, promoting progradation and aggradation, the primary depositional components of normal regression. This condition commonly persists during the early stages of sea level fall. Thus, the lower boundary is the maximum flooding surface; prograding clinoforms will downlap the MFS. The landward part of the upper boundary includes the subaerial unconformity, and offshore the basal surface of forced regression; clinoforms will terminate below these surfaces (toplap). In standard depositional sequence models, the upper surface is a sequence boundary. The HST underlies the FSST and overlies the TST. Depositional systems that make up the HST can include fluvial, delta, barrier island – lagoon, coastal, and shelf deposits.
Falling stage systems tracts (FSST)
During sea level fall, the rate of change increases to the point where it exceeds sedimentation rates, whereupon the shoreline and shoreface are forced towards the shelf-platform margin. Thus, the shoreline trajectory downsteps seaward in concert with each regressive wedge. The FSST is bound at the base the basal surface of forced regression; at the top by a subaerial unconformity that progresses basinwards during regression, and its correlative conformity beyond the shelf margin. Depositional systems include the shoreface and deeper shelf, plus sediment that bypasses and accumulates beyond the shelf edge (e.g. base of slope).
The FSST is also known as the forced regression systems tract.
Lowstand systems tracts (LST)
During the early part of sea level rise, sediment is still being delivered to the shelf (i.e. sedimentation rates are greater than baselevel change). Sediment that enters the base of slope and deep basin settings will be deposited as submarine fans and channels over the subaerial unconformity and its correlative conformity (that developed during the FSST); this is where Hunt and Tucker (1992) place the base of the LST in the depositional sequence stratigraphic model – it is also the sequence boundary. The top of the LST is the maximum regressive surface, that marks the stratigraphic response to the onset of transgression (and end of regression). At this stage of baselevel change, it is argued that sedimentation rates decrease in the deep basin because the source of sediment is significantly diminished by rising river-channel baselevels. Condensed sections may develop if sedimentation decreases significantly.
Low stand depositional systems tend to be dominated by base of slope fans and submarine fans (sediment gravity flows, mass transfer deposits – MTDs), but there will also be correlative shoreline and shoreface systems developed along the landward part of the LST.
Transgressive systems tracts (TST)
The increasing rates of sea level rise and accommodation soon overtake sedimentation rates, initiating shoreline retreat and retrogradational stacking patterns. Clinoforms onlap the maximum regressive surface (MRS) at the base of TSTs; the upper bounding surface is the maximum flooding (MFS). Shoreface ravinement may remove some or all the MRS and subaerial unconformity. Strata that overlie and downlap the MFS are part of the subsequent HST. The TST overlies the HST and, where it is developed, the FSST.
Regressive systems tract (RST)
The transgressive-regressive sequence model, an alternative to the depositional sequence model, was popularized by Embry and Johannessen (1992). T-R sequences have only two systems tracts; a transgressive and a regressive systems tract. The regressive systems tract contains all strata deposited from the beginning to the end of regression; there is no subdivision into normal and forced regression. This approach is particularly sensible when key stratigraphic surfaces, like the basal surface of forced regression, that separates HST and FSST, cannot be identified; it avoids the temptation to assume this surface is present because the model demands it.
The boundaries of an RST are defined by surfaces that have high preservation potential and are relatively easy to find. The base is the maximum flooding surface that is commonly represented by abrupt lithofacies changes (including condensed sections); the top is the subaerial unconformity and in the marine part of the succession, the maximum regressive surface (MRS); this is also the T-R sequence boundary.
The LST is also included in the RST because:
- They both share the maximum regressive surface at their upper boundary, and
- The end of regression does not coincide with the limit of baselevel fall, but at some point during the early stage of sea level rise.
Thus, the RST in the T-R model contains the highstand, falling stage, and lowstand systems tracts. Note that the systems tract diagram above does not portray the RST because the subaerial unconformity underlies the LST in the depositional sequence model, but overlies the LST in the T-R model.
This post is part of the How To…series on Stratigraphy and Sequence Stratigraphy
Other posts in this series on Stratigraphy and Sequence Stratigraphy
Stratigraphic surfaces in outcrop – baselevel fall
Stratigraphic surfaces in outcrop – baselevel rise
A timeline of stratigraphic principles; 15th to 18th C
A timeline of stratigraphic principles; 19th C to 1950
A timeline of stratigraphic principles; 1950-1977
Baselevel, Base-level, and Base level
Sediment accommodation and supply
Autogenic or allogenic dynamics in stratigraphy?
Stratigraphic cycles: What are they?
Sequence stratigraphic surfaces
Shorelines and shoreline trajectories
Stratigraphic trends and stacking patterns
Stratigraphic condensation – condensed sections