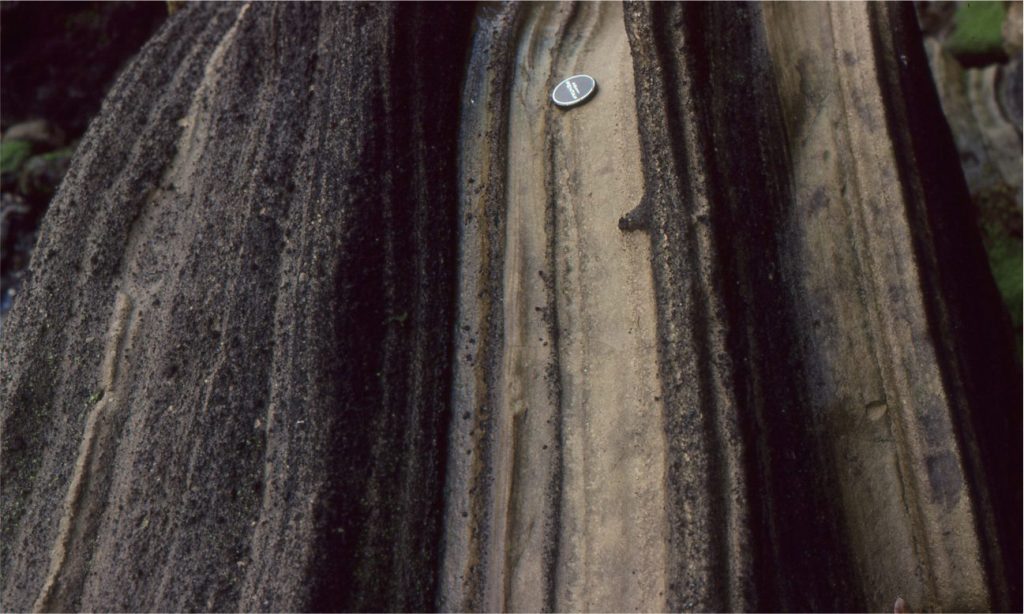
I have been under the (mistaken) impression that accretionary aggregates, including accretionary lapilli, are occasional odd-ball structures borne of explosive volcanic eruptions. Turns out that, not only are they common in volcanic eruptions that produce ash columns (e.g., plinian, vulcanian eruptions) and plumes atop pyroclastic density currents, but are found in the ejecta of large meteorite impacts (e.g. Sudbury impact structure, Huber and Koeberl, 2017; Open Access). Aggregation of fine ash seems to be the norm and, moreover, is probably an important mechanism for dilution and removal of fine ash from billowing, turbulent ash clouds.
Accretionary aggregates are distinguished from other lapilli by:
- Their spherical to oblate form, commonly 2-25 mm in diameter.
- Where present, concentric layers may be continuous or locally discordant.
- Aggregate cores may consist of ash or a lithic fragment, usually juvenile pumice or more dense fragments of lava.
- Ash in the aggregate core is commonly coarser grained than that on the rim.
Concentrically layered accretionary lapilli are the most recognizable, but they are part of a spectrum of aggregate forms that includes completely unstructured types. Brown et al., (2010; PDF available) have identified five distinct types based on earlier classification schemes and their own examination of ignimbrite and co-ignimbrite ash deposits on Tenerife, summarized in the diagram below.
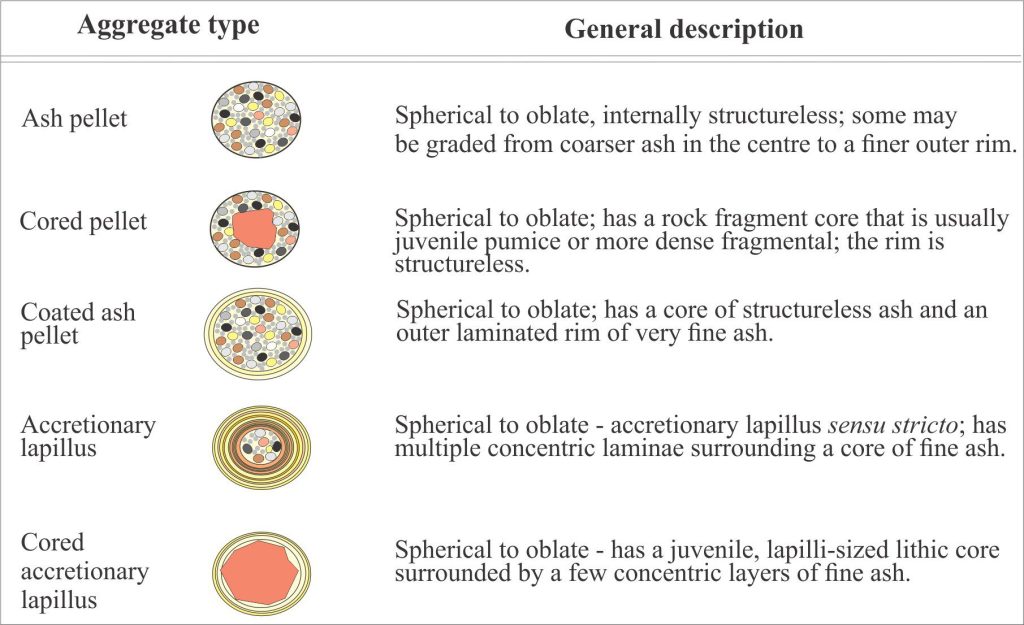
How do accretionary aggregates form?
Eruption columns and plumes consist primarily of juvenile fragmental material and a gas phase that consists of water vapour and subordinate CO2 and other volatiles. The volatiles are derived from the original magma, and in some eruptions from groundwater or sea water (particularly phreatomagmatic eruptions). Particles in the eruption column are supported by turbulence, which also means they will be constantly tumbling. Turbulence also acts as an engine that generates electrostatic charges on ash particles – we know this because lightning frequently discharges within eruption clouds. Aggregate accretion is the happy marriage between frequently colliding, electrically charged ash particles and water bonding. Freezing may also occur in columns that penetrate the upper atmosphere.
A useful and more familiar analogue for this process is the formation of hail, or hailstones during thunder storms and tornadoes. The diagram below describes the accretion of supercooled water droplets and small ice crystals to a hailstone as it spins in the updraft of a cumulonimbus cloud. The ice crystals develop positive electrostatic charges in the upper part of the cloud and the growing hailstones negative charges in the lower part of the cloud – hence the electrical discharges. The hailstone analogy seems reasonable given the similar geometric structures of hail and accretionary lapilli, the role of electrostatic charges, and the violent, turbulent movement of particles that sustain both storm clouds and eruption columns.
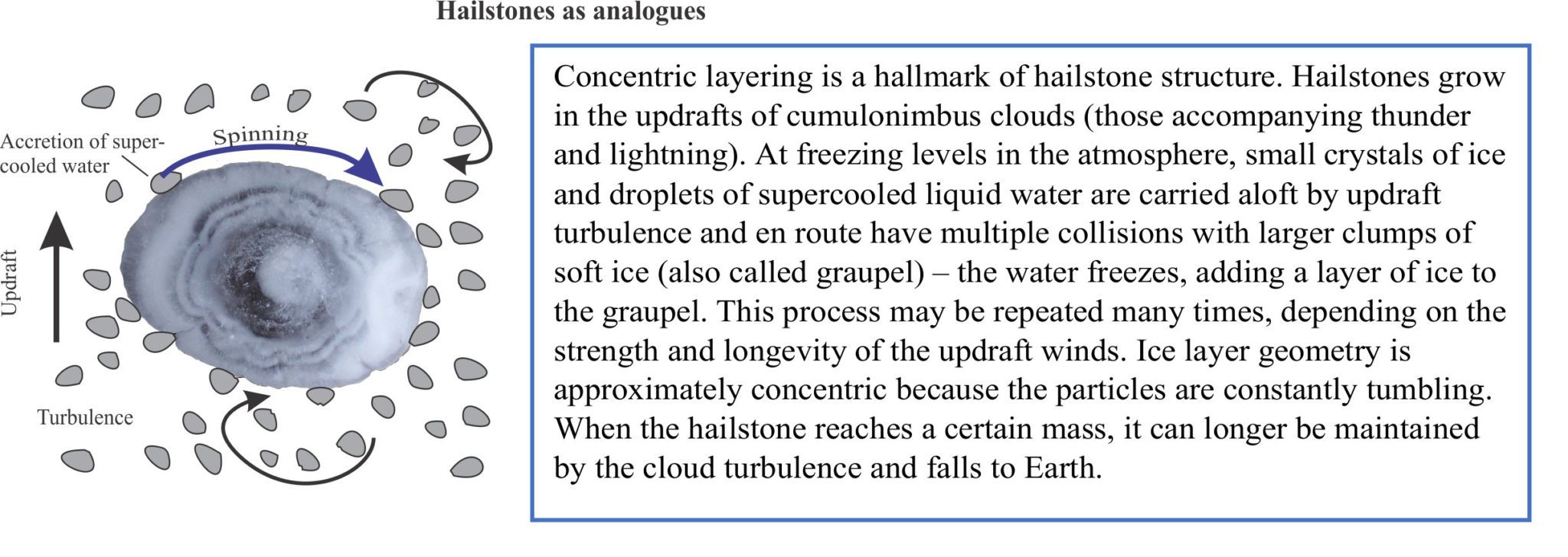
Like hail, volcaniclastic aggregate size is probably dependent on the amount of time spent tumbling through an eruption cloud. At some point the aggregates will be too heavy to be maintained by the column turbulence and will fall, along with other fragmentals of similar density and size. Their preservation upon sedimentation will depend on how tight the aggregate particles are bound. Indeed, the preservation potential of many aggregates is probably low if the only mechanisms holding them together are static electricity and a film of water. Aggregates that are frozen at high elevations will likely disaggregate as they fall through warmer air. However, many accretionary aggregates, particularly accretionary lapilli (those with concentric layering) are preserved intact, deformed by compaction, or broken (i.e., brittle behaviour) which implies they are significantly more robust, inviting the suggestion that an additional particle bonding process operates during formation.
Experiments on aggregate binding
One possible mechanism involves rapid precipitation (in the eruption cloud) of sulphate and chloride salts, and that these precipitates cement aggregate particles. Experiments to test this hypothesis were conducted by Mueller et al., 2016, using a fluidized bed containing small glass beads and natural volcanic ash. Fluidization was maintained with a flow of hot air. Loosely bound aggregates formed when water vapour was introduced to the air stream but their preservation during sedimentation was low. The number of aggregates formed in this way increased with increasing humidity. Preservation of aggregates was only achieved when NaCl solution was added to the air stream, coating the beads and ash particles. Examination of the aggregates showed that small NaCl crystals had precipitated at particle contacts within the fluidized bed (i.e., prior to deposition) – in effect, acting as cement. Both Na and Cl are common dissolved constituents in aerosols and the water-vapour phase of eruption columns (in addition to other halides and sulphates), lending credence to the experiment results.
Some examples
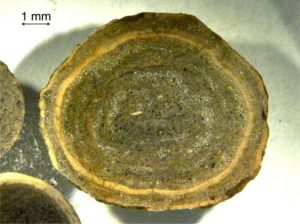
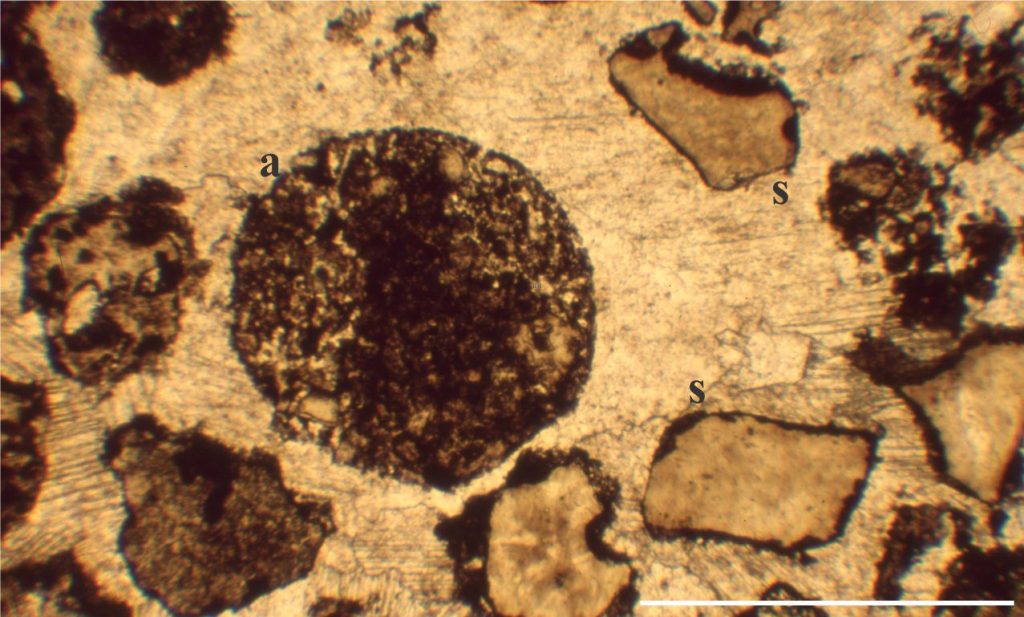
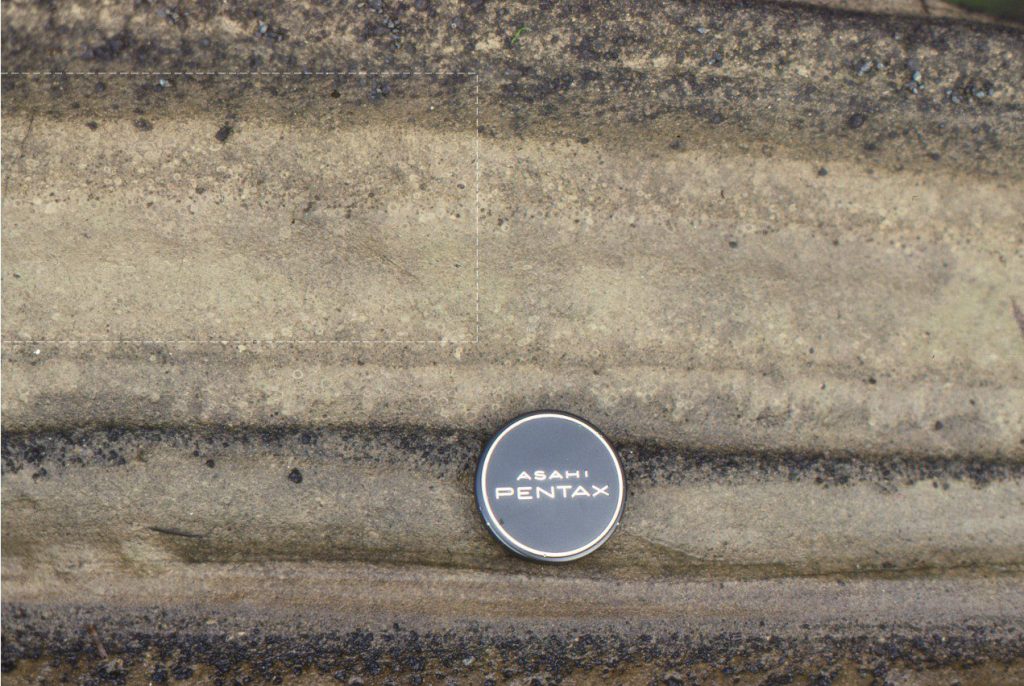
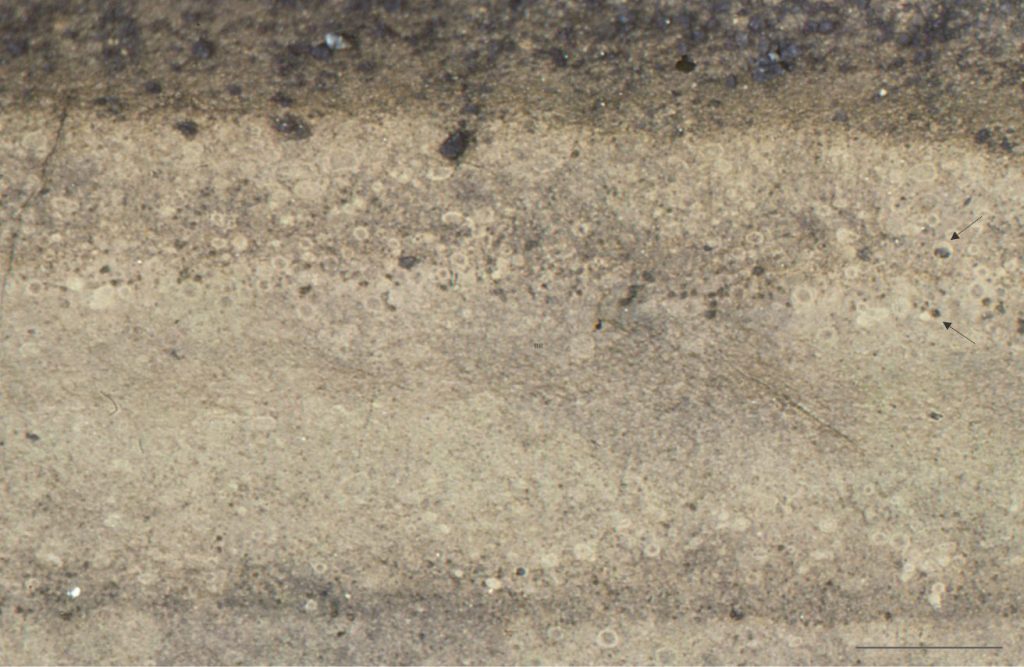
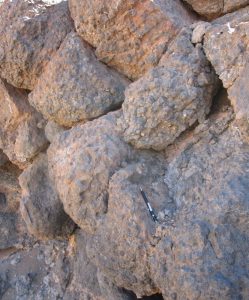
Coated ash pellets
The two image pairs below show coated ash pellets (aggregates) in the Late Miocene – Pliocene Minden Rhyolite, a stratigraphic unit associated with caldera collapse, rhyolite dome extrusion, and PDCs including partly welded to non-welded ignimbrites and pyroclastic surges. Caldera formation was part of the Miocene-Pliocene Coromandel Volcanic Zone – an older cousin to Taupo Volcanic zone, eastern New Zealand. The northern limit of the caldera structural boundary coincides with Whitianga township. These outcrops are located at the north end of Hahei Beach, eastern Coromandel.
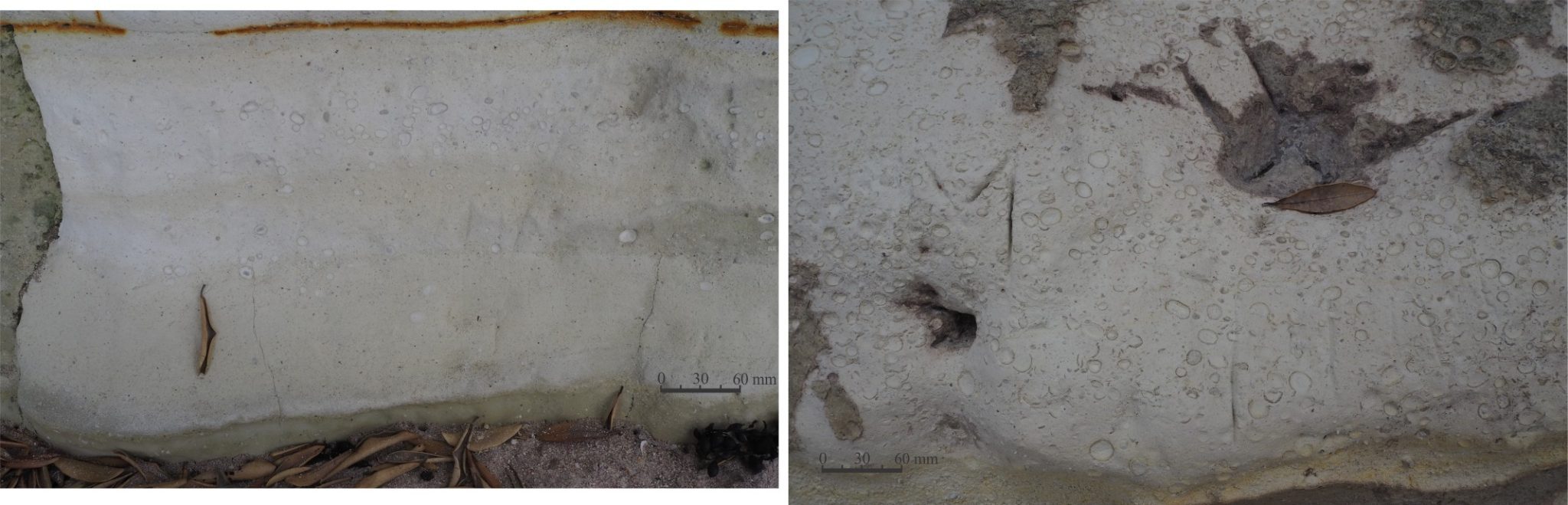
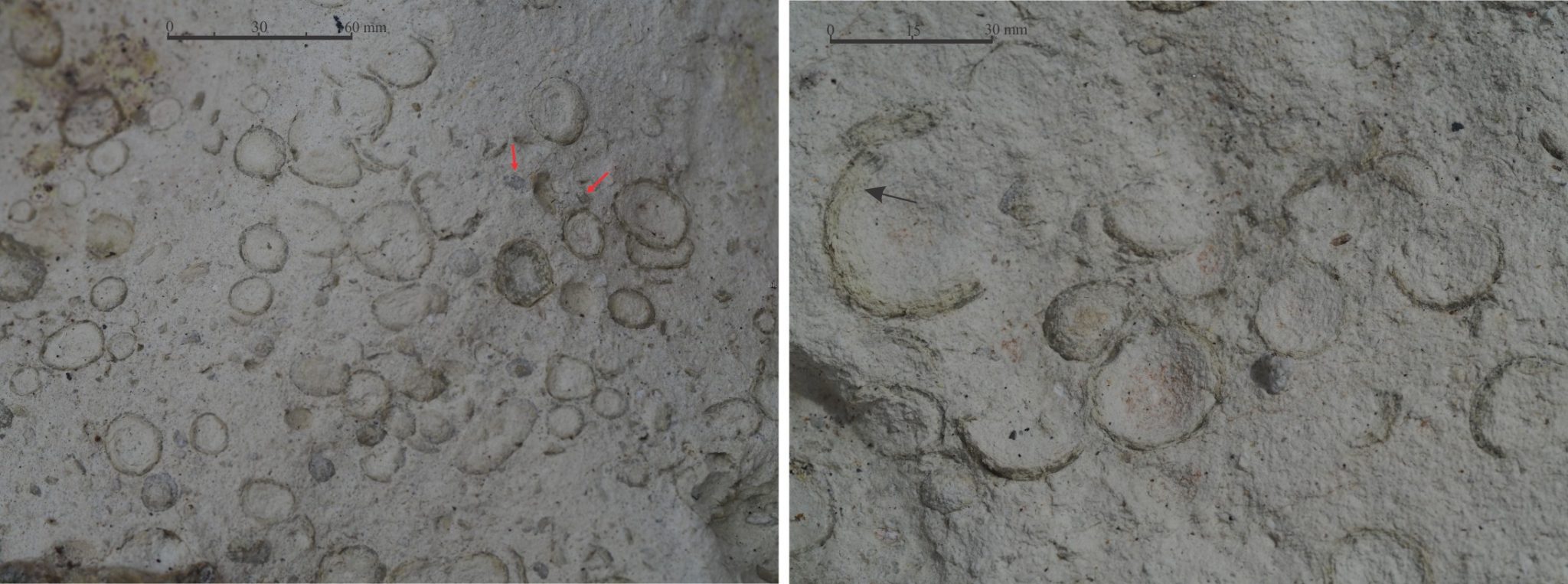
Related posts
Ignimbrites in outcrop and thin section
Volcanics in outcrop: Pyroclastic density currents
Volcanics in outcrop: Secondary volcaniclastics