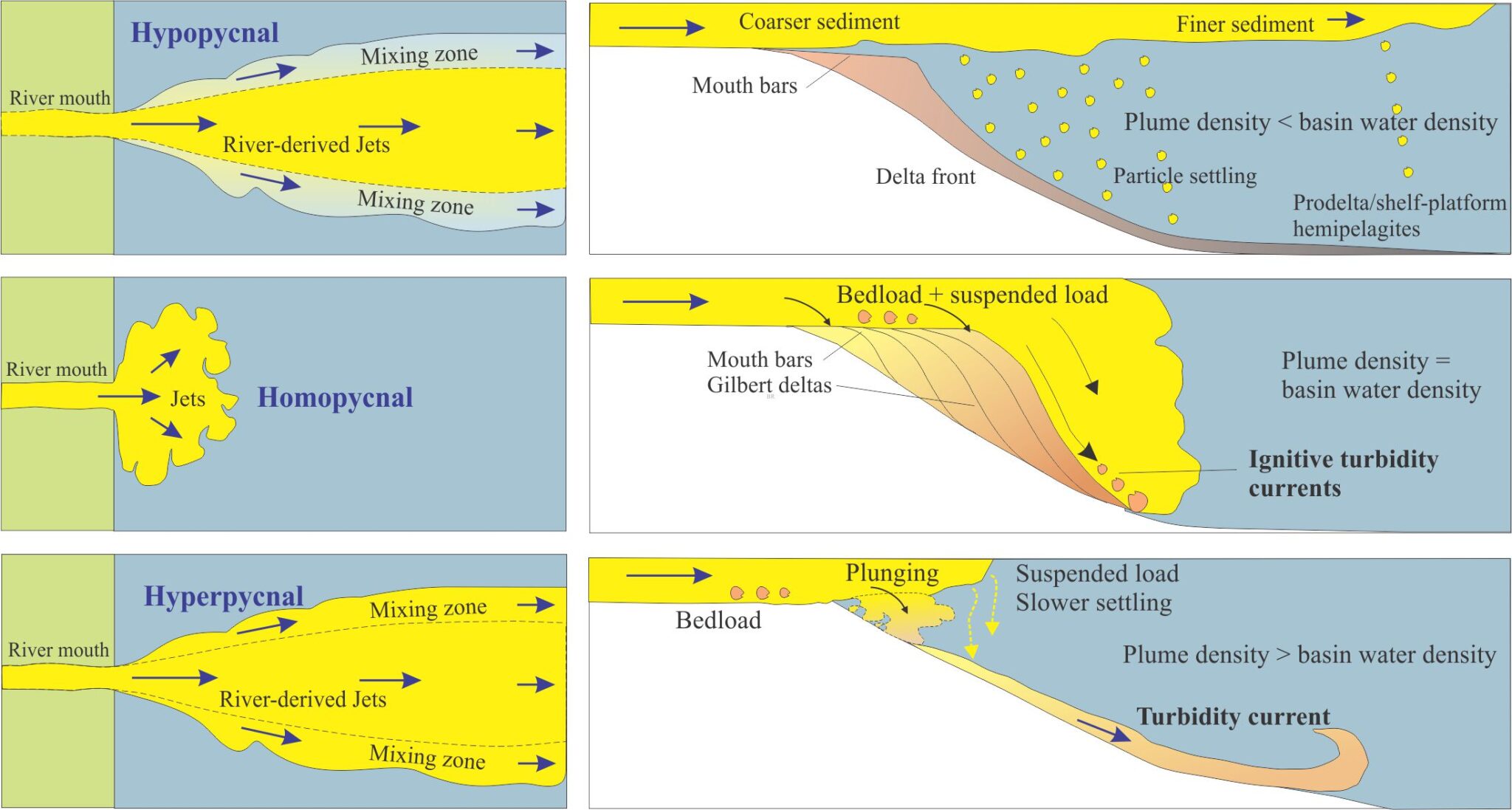
The delivery of sediment to sedimentary basins
It is estimated that 95% of the terrigenous sediment load supplied to sedimentary basins is delivered by rivers (J. Syvitsky, 2003). Although rivers discharge some fine suspended sediment during non-flood flow (e.g., clays, organic debris), it is during periods of flooding that most sediment is carried across basin margins, predominantly as suspended loads. Fluvial processes cease to be the sole operators at the point of channel entry into a standing body of water (lake, ocean). In marine settings, sediment is redistributed by processes that include tidal and wave-generated currents, and different kinds of mass transport deposits (MTDs) and sediment gravity flow.
The manner in which the sediment load transits the freshwater – seawater divide will in large part determine how and where it is distributed – will sediment be retained near the shoreline, for example as a delta, or will it be distributed across the shelf? Or will sediment move through submarine canyons or shelf-edge gullies, bypassing the shelf en route to base-of-slope and deep basin submarine fans?
Formation of sediment plumes at river mouths is a common occurrence during flood events that result from storm precipitation, spring thaw, and natural or artificial dam breakouts (e.g., jökulhaups). Plume behaviour depends on factors such as sediment concentration and plume density, and importantly, the contrast in density between the freshwater plume and water body. Charles Bates (1953) used the analogy of river mouth jet flow as the primary mechanism for sediment delivery and delta formation (his analysis is still quoted regularly). The dimensions of the jet correspond to those of the channel at the river mouth; the jet is characterised by turbulent flow. River mouth jets feed suspended sediment to the plumes and move coarse-grained sediment as bedload across the river water-body divide. Flow is unconfined beyond the river mouth.
Bates defined three types of plume behaviour based on the density contrast between the plume and the receiving lake water or seawater: hypopycnal, homopycnal, and hyperpycnal flows. We can make some general statements about plume dynamics:
- Flow velocity and the degree of turbulence decrease with plume expansion because of frictional losses at plume boundaries and mixing with ambient water in the lake or sea.
- In all cases the initial density of the plume fluid phase is that of freshwater, but this may vary depending on temperature, for example seasonally cold waters during spring thaw entering a warmer lake or sea.
- The bulk density of a plume depends primarily on the concentration of suspended sediment.
- The maximum suspended grain size in a plume is probably fine-grained sand.
[…pycnal is from ancient Greek puknos, meaning dense, compact, or thick]
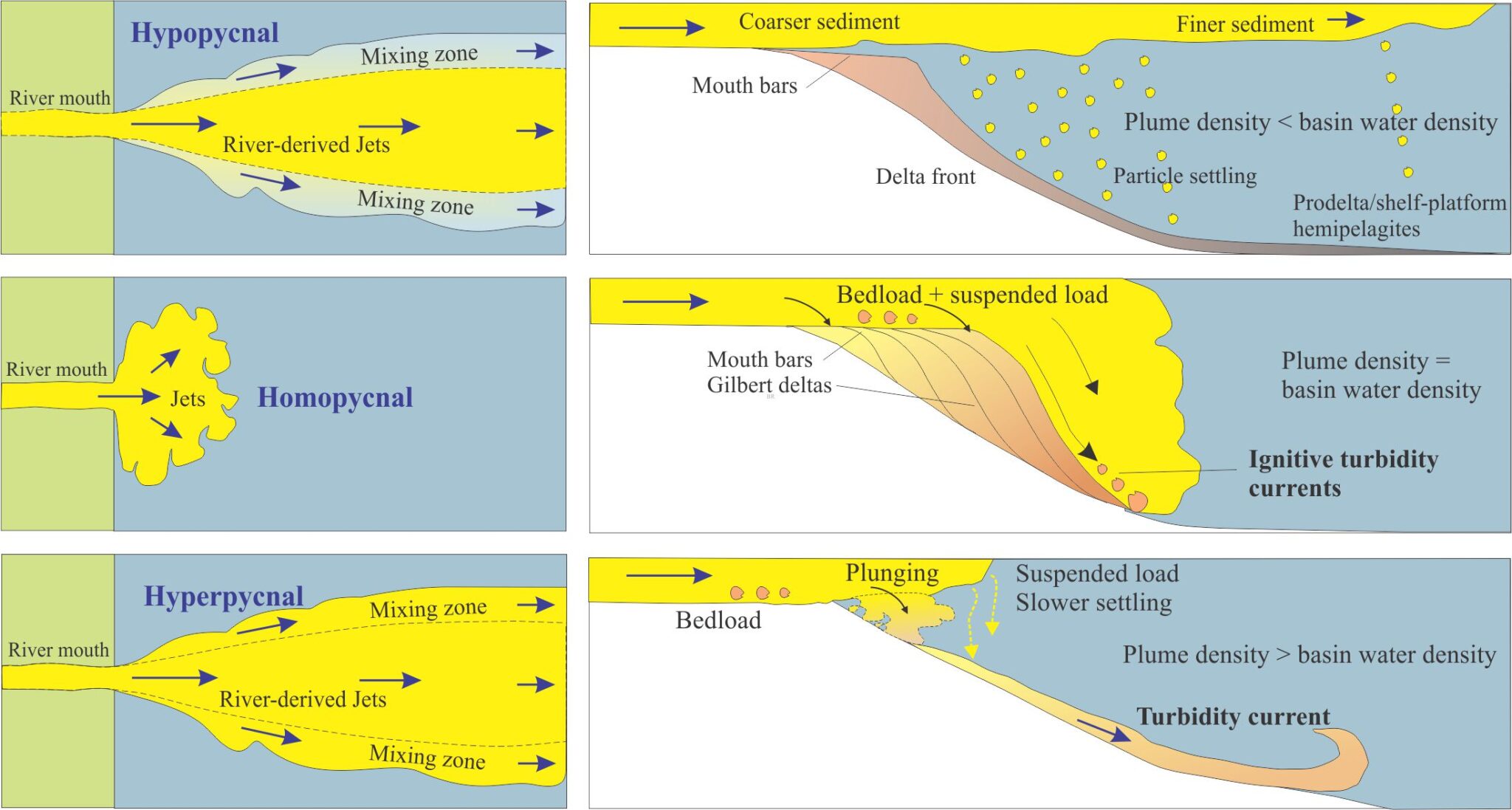
Hypopycnal flow
When plume density is less than the lake or sea the plume will be buoyant and will tend to disperse across the top of the water body. According to descriptions by Mulder et al., (2003) and Zavala (2020, open access), relatively coarse sediment will fall rapidly out of suspension close to the river mouth forming mouth bars, and finer-grained sediment progressively farther from shore – the latter will form laminated hemipelagites or prodelta deposits. Particle settling velocities will depend on particle density, size, particle shape, and the degree of turbulence. Stokes Law will apply where the influence of jetting turbulence is minimal.
It is difficult to envisage hypopycnal flows developing in lakes unless the river water is warmer than the lake water. If water temperatures are about the same, then any sediment in the plume will render it denser than the lake water and the plume will not be buoyant.
Hypopycnal flows are more likely to form in marine basins although even here, the plume density must be less than that of the ambient seawater that in temperate to tropical latitudes ranges from about 1.027 to 1.024 kg/m3.
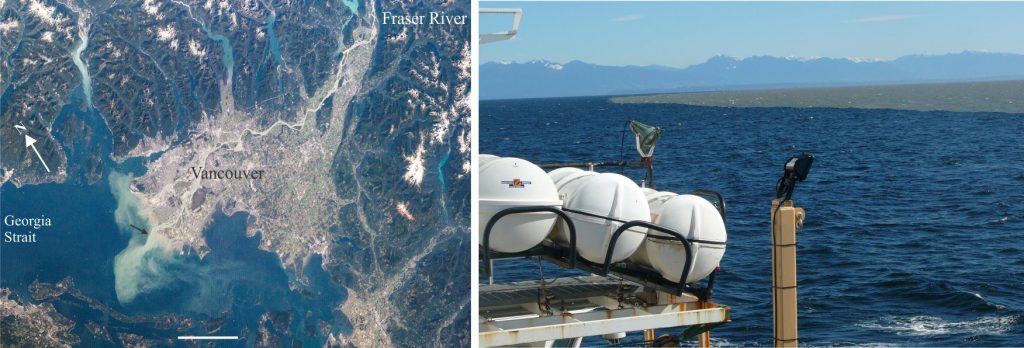
Homopycnal flow
Homopycnal flows form when the density of riverine water masses is about the same as that of the receiving water body (i.e., the density contrast approaches zero). In this situation, the plume momentum diminishes abruptly and most of the sediment accumulates in delta-like mouth bars, the adjacent delta slope, or Gilbert delta foresets. In some cases, jetting may be powerful enough to move coarse-grained bedload as crossbedded dune bedforms, erosional scours, climbing ripples, and even antidunes that indicate supercritical flow (Winsemann et al., 2021, open access). These deposits can potentially be reworked by nearshore processes. Instabilities caused by rapid sediment accumulation on the delta front will result in sediment cascades and ignitive sediment gravity flows.
The finest and least dense sediment fraction of these riverine plumes (clays, plant debris) will mix with the ambient lake or seawater, will remain in suspension for longer periods, and have much lower settling velocities (an expression of Stokes Law). This residual plume may extend well beyond the delta front.
[N.B. The term ignitive refers to sediment gravity flows, principally turbidity currents, that form from pre-existing deposits, and are triggered by processes such as slope failure, seismicity, and canyon-margin collapse, or transform from debris flows. Ignition in this sense means flow acceleration and entrainment of sediment that produces what G.Parker (1982) refers to as a “self-sustaining turbidity current.]
Hyperpycnal flow
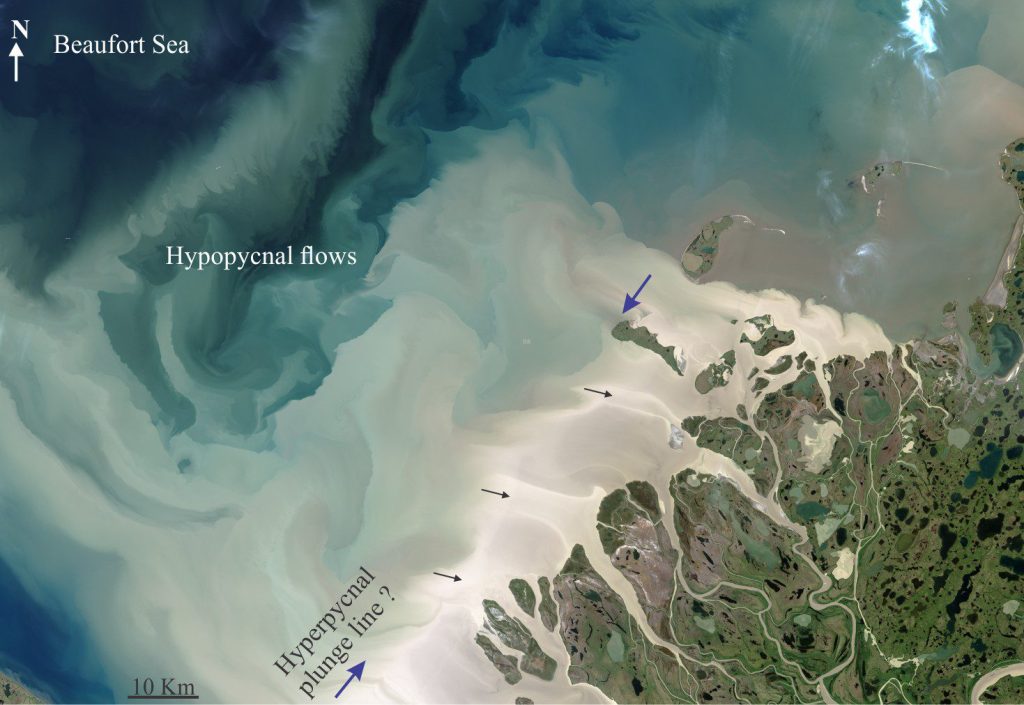
A hyperpycnal flow develops when the density of a fluvial plume is greater than that of the receiving water body – more attention has been paid to this category of flow than the other two. Hyperpycnal flows can form in lacustrine and marine environments. The general theory, based on observations in modern rivers, flume experiments, and modelling, is that the dense plume (freshwater plus sediment) will collapse soon after entry and suspended sediment will plunge towards the sea or lake bed. The rate at which sediment plunges from the plume will depend on the initial plume density, the degree of turbulence generated by the river-mouth jet, and the development of convective instabilities in the plume (e.g., Parsons et al., 2001 PDF available).
Based on observations of modern plumes, Mulder et al., (2003, ibid) comment that plume densities must be >36 kg/m3 for plunging to take place. although much lower densities are also possible, as demonstrated in flume experiments by (Parsons et al. op cit.). The experimental plumes in this case were hypopycnal having densities less than those normally found in hyperpycnal plumes. The experimental density contrast resulted in downward-projecting convective fingers of sediment that “spawned” hyperpycnal flows (turbidity currents) – shown diagrammatically below.
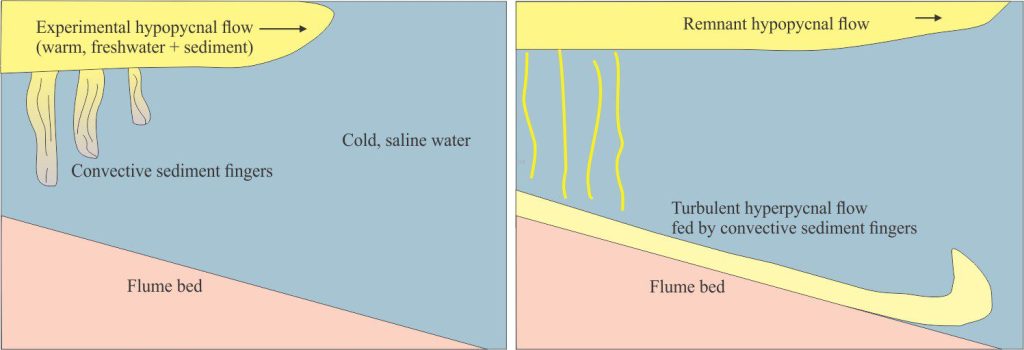
Plunging sediment columns can form two main types of deposit:
- Sediment remains on the sea floor. The deposit may show normal grading as coarser material falls from suspension faster than finer-grained sediment. These deposits are prone to reworking by nearshore currents and waves, or as sediment gravity flows if depositional slopes fail, for example as turbidity currents. This kind of flow is NOT a hyperpycnal flow.
- Hyperpycnal flows develop when the plunging sediment plume or convective sediment fingers initiate bottom-hugging flows. The resulting deposit is called a hyperpycnite. The bottom-hugging flows are considered to be turbulent and the deposits those of relatively dilute turbidity currents.
- Hyperpycnal turbidity currents will be maintained until their density becomes less than the ambient water mass. Deposition of sediment from turbulent suspension will reduce flow density, but ingestion of seawater, particularly through the flow head, will tend to increase the density (replacing freshwater). However, flow density will eventually lessen and the flow may separate from the sea or lake bed and continue to move within the water mass.
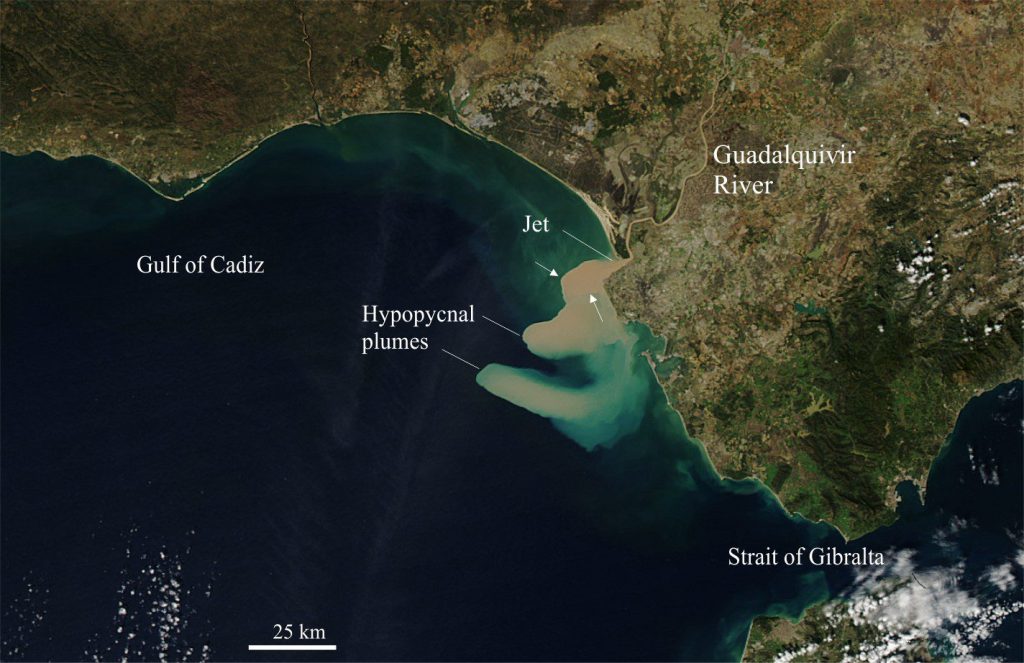
Comparing hyperpycnal flows and ignitive turbidity currents
Important differences between hyperpycnal flows and ignitive turbidity currents include:
- Hyperpycnal flow velocities depend on the momentum generated as sediment plunges. Thus, there is an initial velocity that decreases as sediment is deposited and water is ingested into the flow, both processes decreasing flow density and turbulence.
- Turbidity currents generated by ignitive processes have higher initial momentum (because of the trigger mechanisms) and accelerate, or ignite downslope.
- Hyperpycnal flows are generally more dilute than ignitive turbidity currents.
- Hyperpycnal flow velocities are generally lower than those of ignitive sediment gravity flows.
- A typical ignitive turbidity current will generate normal grading and a well-known sequence of sedimentary structures that form with varying contributions from bedload and suspension load transport, and evolving conditions of flow density and velocity, including in some flows the transition from supercritical to subcritical conditions. This type of turbidity current commonly generates an eroded base.
- In comparison, Mulder et al., (op. cit.) argue that a typical hyperpycnite will contain a lower reverse-graded bed (as plume density increases in concert with the storm peak flow), overlain by a normal graded unit deposited as the storm flow wanes. The two graded units may be separated by a surface of erosion.
- Plink-Björklund and Steel (2004) suggest that sustained hyperpycnal flow turbidites are preserved as ungraded or poorly graded sandstones, commonly with small traction structures (e.g., ripples, small dunes).
- Talling (2014) on the other hand suggests that the slower, more dilute hyperpycnal flows will generate millimetre- to centimetre-thick laminated deposits.
- Hyperpycnal flows may last for hours or days depending on the severity of the storm and the continued supply of sediment to the plume. Prolonged plume activity can potentially deposit hyperpycnites more than several metres thick.
- Turbidity currents probably have much shorter duration, are capable of forming deposits from a few millimetres to several metres thick, and run-out distances that can extend 10s to 100s of kilometres.
- River jetting during prolonged floods (including spring thaws that can last days or weeks) may produce a series of overlapping plumes rather than a single plume. In this case, the resulting hyperpycnites would also overlap laterally and vertically. An example of multiple hypopycnal and hyperpycnal plumes associated with the Copper River spring thaw, overprinted by tidal fluctuations, is shown below.
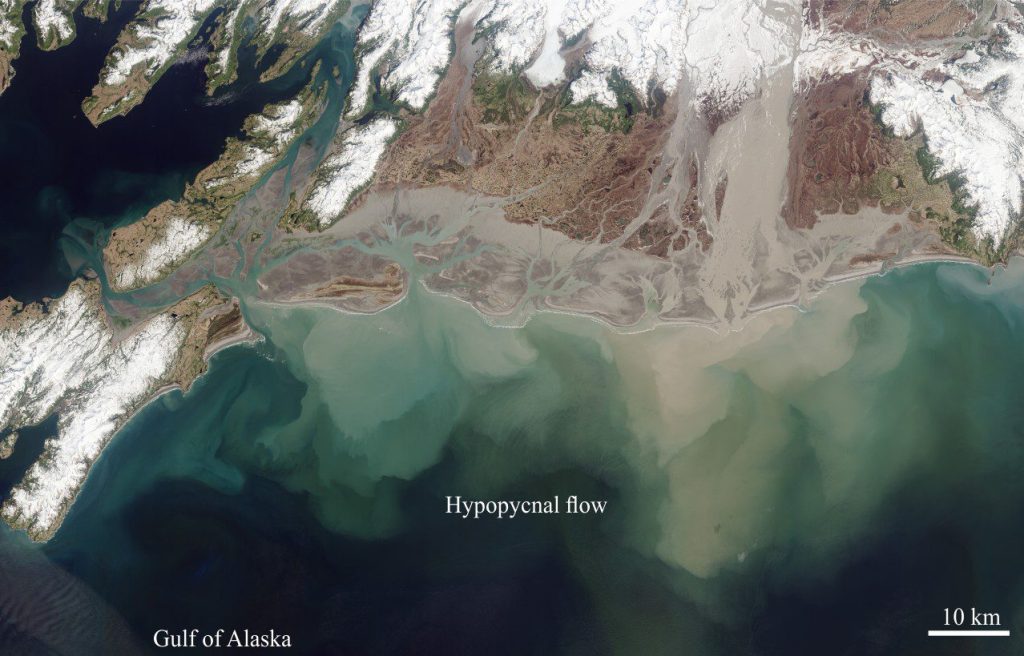
Differentiating hyperpycnites from (ignitive) turbidites
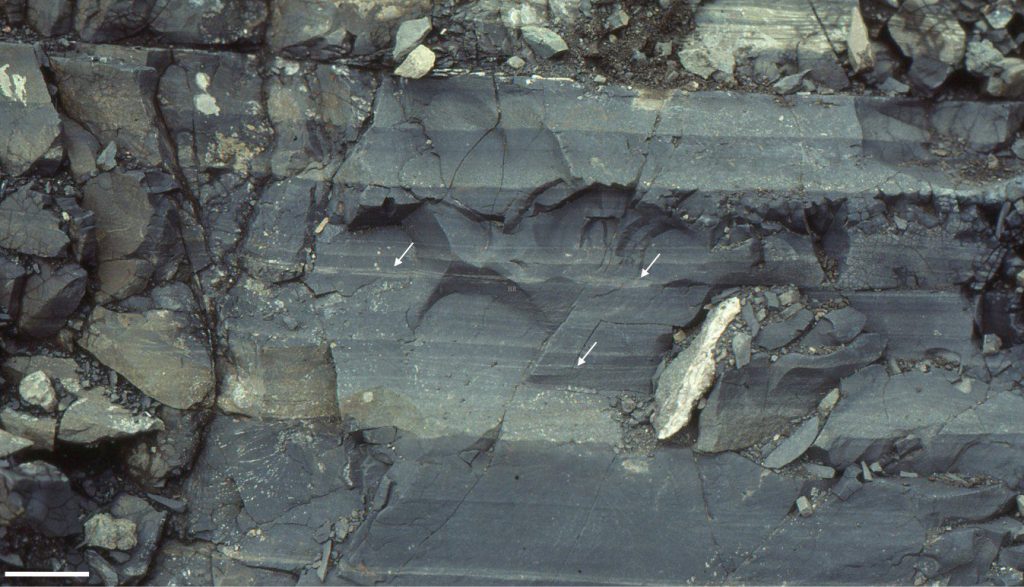
There are very few direct observations of hyperpycnite deposition – Mulder et al., (2003, op. cit.), Talling (op. cit.), Shamughan (2018a), and Zavala (op cit.) provide details on some examples, inferred from modeling and observed in some modern settings.
Shanmugam (2018b, open access) has opined that it is not possible to make the distinction given our present state of observations. Mulder et al., have presented their ideal hyperpycnite (inverse followed by normal grading), while Talling’s typical depositional unit consists of thin laminated beds (illustrated below).
In one of the more convincing examples, Plink-Björklund and Steel (op cit.) have demonstrated a direct facies link between fluvial and turbidite-bearing shelf-edge channels by mapping and tracing beds down-dip over several kilometres. The turbidites, interpreted to be the product of sustained hyperpycnal flows, are ungraded or poorly graded sandstones that contain abundant plant material and small bedforms indicative of traction.
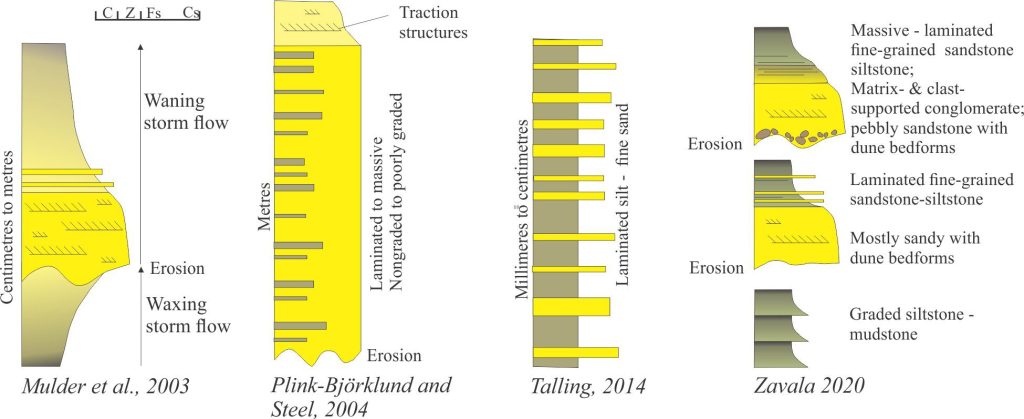
Zavala (op cit.) provides details of several bedding types and bedforms, including a range of lateral facies changes. Zavala’s descriptions are based primarily on ancient examples and include varying degrees of clast- and matrix-supported deposits that include cohesive, gravelly debris flows, imbrication, beds containing mudstone intraclasts, various dune bedforms and climbing ripples, laminated deposits, and hummocky cross-stratification (HCS).
Zavala’s contention is that the features he recognises as typical of hyperpycnites are frequently misinterpreted as turbidites (or debris flows). Indeed, many of the bed types and bedforms are described from turbidites. His hyperpycnites cover the gamut of sedimentary structures, sediment compositions, and flow rheologies (cohesive and plastic, to non-cohesive and Newtonian) that have been described in countless examples of ignitive debris flows and turbidity currents. Furthermore, bedforms like parting lineation require supercritical flow conditions – some of the dune bedforms he illustrates may be antidunes, that also require supercritical flow. Given that many authors consider hyperpycnal flows to be relatively slow moving, it is uncertain whether such supercritical conditions will develop.
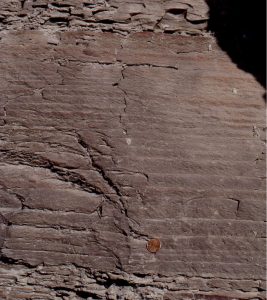
The existence of hyperpycnal flows and recognition of their (probable) importance as distributors of sediment across deltas, shelves, and platforms is acknowledged. However, there is a fair bit of ambiguity in the suite of bed types, bedforms, their lateral and vertical variations when we compare hyperpycnite turbidites and ignitive turbidites. Perhaps one of the best ways to demonstrate hyperpycnite flows in the rock record is to do what Plink-Björklund and Steel (2004) have done – trace individual beds and packages down depositional dip from fluvial channels to submarine channels. However, even here it may not be possible to unambiguously exclude mechanisms that produce turbidity current ignition.
For the time being, there are several good models to choose from.
Other posts in this series on lithofacies
Sandstone lithofacies
Sedimentary lithofacies – An introduction
Ripple lithofacies: Ubiquitous bedforms
Ripple lithofacies influenced by tides
Tabular and trough crossbed lithofacies
Laminated sandstone lithofacies
Low-angle crossbedded sandstone
Hummocky and swaley cross-stratification
Lithofacies beyond supercritical antidunes
Subaqueous dunes influenced by tides
Gravel lithofacies
Introducing coarse-grained lithofacies
Crossbedded gravel lithofacies