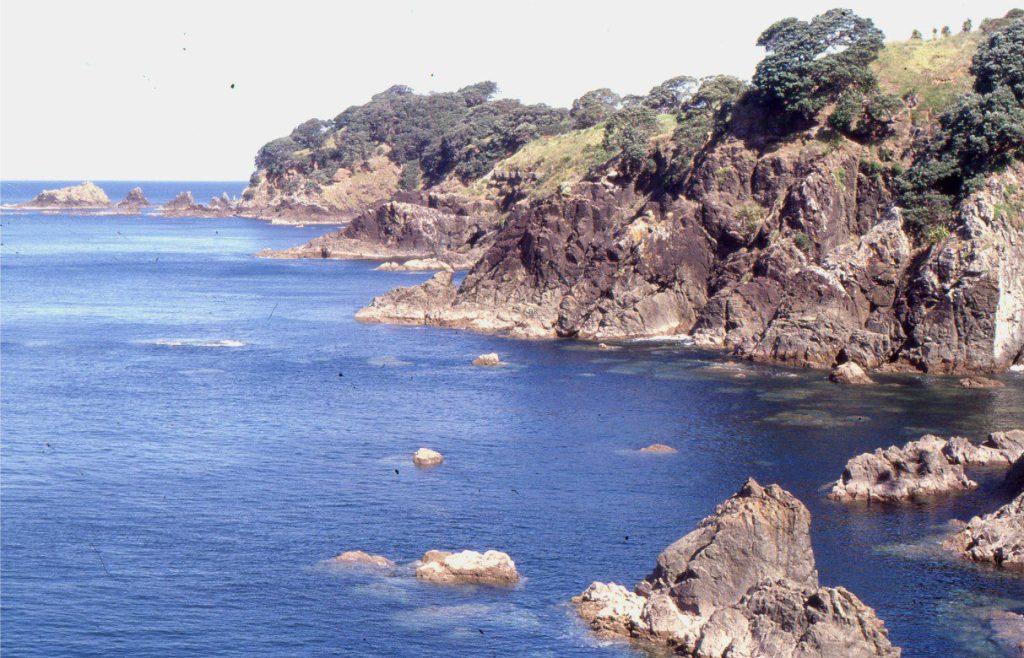
Pebble, cobble and boulder beaches and their shoreface equivalents.
The swimmability of a beach, if it was a popularity contest, is primarily a function of its wave climate and sandiness. A decent surf can be fun, notwithstanding the inevitable rip currents, but subdued waves are probably safer. A sandy beach is a lot easier on the feet (notwithstanding the heat in black sand exposed to the sun); pebble, cobble, and boulder beaches are less conducive to that mad-dash into the waves, particularly when clasts are encrusted with oysters and barnacles.
However, gravel beaches are more interesting from a (biased) sedimentological perspective. They elicit questions like:
- Where did all the cobbles come from?
- How are they moved across the beach?
- Is the energy required for clast entrainment available in the current environmental conditions or are they relics of some past event?
- Do the gravels extend to the adjacent shoreface and if so, how did they get there?
Consideration of the first question will often lead to answers to other questions. A few scenarios related to modern coastal deposits are listed below. Some of these will apply to ancient examples.
- First cycle gravel derived from bedrock exposed along the coast, eroded and weathered by coastal processes (waves, precipitation, freeze-thaw, plant roots, salt crystal expansion).
- First cycle gravel sourced directly from terrestrial drainage basins and transported by rivers, or across alluvial fans and fan deltas that drain to the coast.
- Second cycle gravel derived from older deposits exposed at or near the coast. Examples include older fluvial, alluvial, glaciogenic, or volcaniclastic conglomerate and breccia.
- First or second cycle gravel moved across a shelf during sea level lowstand and subsequently stranded there once sea level rises. These deposits are palimpsest and available for reworking on the shoreface and beach.
First cycle here refers to the bits of eroded or weathered bedrock that are delivered to a depositional sink, in this case a beach or shoreface. En route the fragments may be rounded and sorted.
Second cycle gravel clasts are derived from older conglomerates or breccias that formed in unrelated depositional systems. For example, gravels sourced from a glacial till and deposited on a beach would be considered second cycle. But gravels reworked from floodplains into adjacent braided channels would be considered first cycle because they are part of the same depositional system and only stored temporarily on the floodplain.
Beach and storm ridge gravels
Most modern, active gravel beaches are located on high energy coasts exposed to constant wave attack. Clast size is highly variable and depends on the composition and fabric of bedrock in the case of first cycle gravels, and on inherited clast size, shape, and stratigraphic distribution in second cycle gravels. Thus, clasts derived from homogenous bedrock will be less prone to breakage and size diminution than those derived from bedrock that contains strong sedimentary or structural fabrics.
Gravel beach slopes are usually steep, >5o; beach grade usually steepens towards its landward extent. Gradients also increase with clast size and wave steepness. There is a general tendency for clast size grading up the beach face, where clasts are largest near the low tide zone, becoming finer up the beach, but there are many exceptions to this where the trend is interrupted by storm waves and surges. Size grading may be less pronounced up narrow beaches. Clast size grading can also occur along the length of a beach – coarser grained at either end, finer in the middle, although smaller pocket beaches (beaches between closely spaced rocky promontories) are less likely to exhibit this trend.
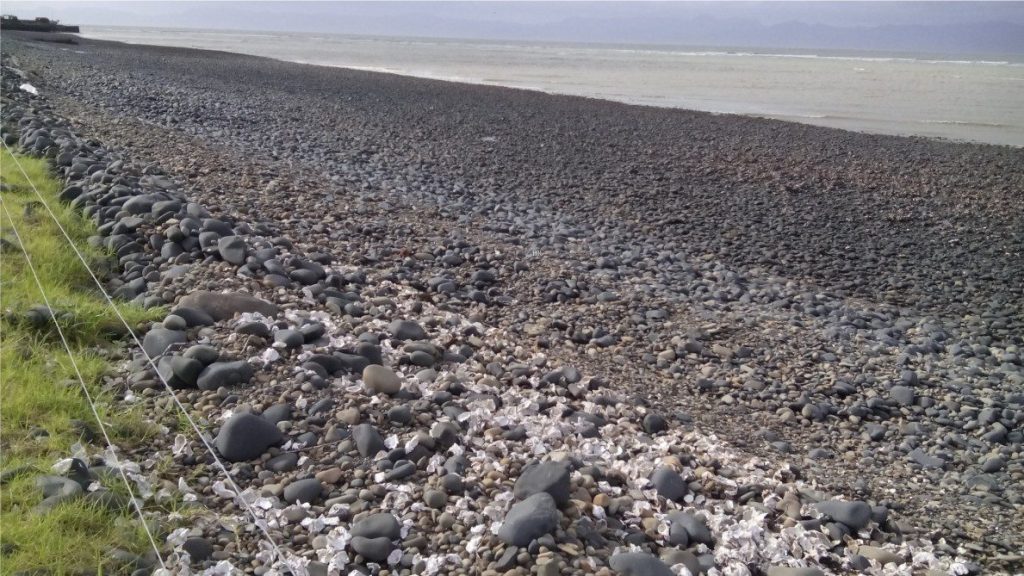
Invertebrate shells and calcareous frameworks like bryozoa, coral, and calcareous algae are common in beach gravels, but they tend to be broken or seriously abraded. Shell fragments may be trapped between larger clasts. Large clasts that move infrequently may be encrusted with by bryozoa, barnacles, calcareous algae (including rhodoliths), and seaweed.
Storm waves move gravel from the shallow shoreface to the beach and beyond the high or spring tide limit onto storm ridges or berms. The ridges are low amplitude mounds, a few centimetres to decimetres high. They generally have gently rounded surfaces on the seaward margin but may be steeper landward. Shelly material tends to be broken. Low tide and high tide berms may also develop, the latter associated with the fairweather limit of wave swash.
The most common lithofacies are low-angle and tabular crossbeds, and massive bedded gravels.
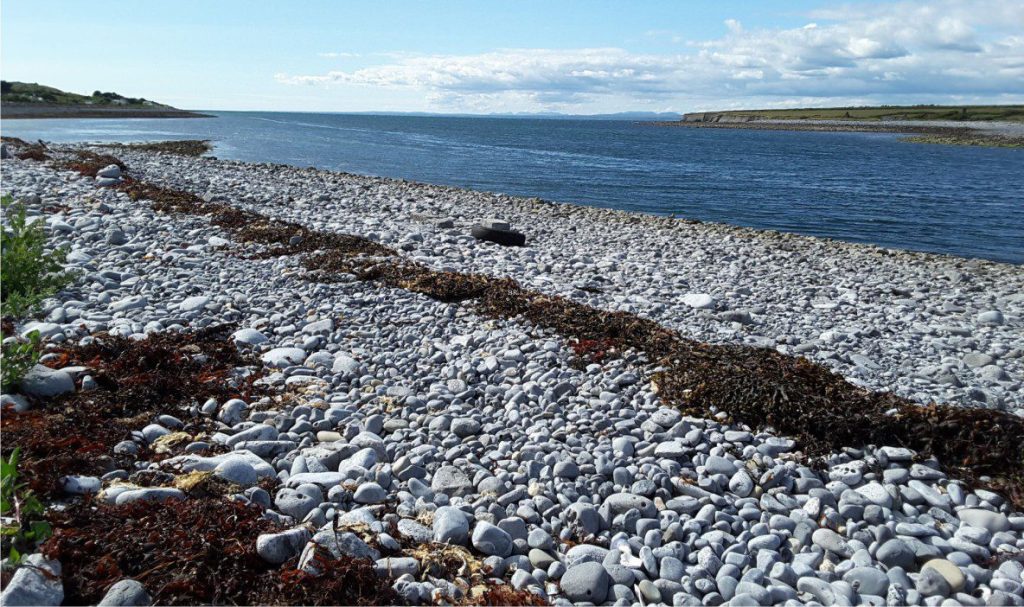
Massive-bedded gravel lithofacies
Cobble and boulder beaches on will seldom have recognisable stratification. They tend to be poorly sorted because smaller clasts (and shells) are trapped in the framework interstices. Massive bedding may pass laterally to low-angle crossbedding where there is a concomitant decrease in grain size (parallel to and up the beach face).
The internal organisation of clasts in storm ridge gravels, regardless of their grain size, tend to be massive because there is little opportunity for reworking and sorting. However crude tabular crossbedding can develop on the landward margins of the ridges if the landward dipping lee faces are steep enough to generate clast cascades.
Low-angle crossbedded gravel lithofacies
Low-angle stratification in fine gravels (mostly pebble grades) forms in the swash-backwash zone. The crossbedding is like that formed in sand although the laminae and crossbed set boundaries tend to be more diffuse (because of grain size). Foreset dip and crossbed set truncation angles are usually less than 10o. Crossbedding is more definitive in pebble-sand mixtures.
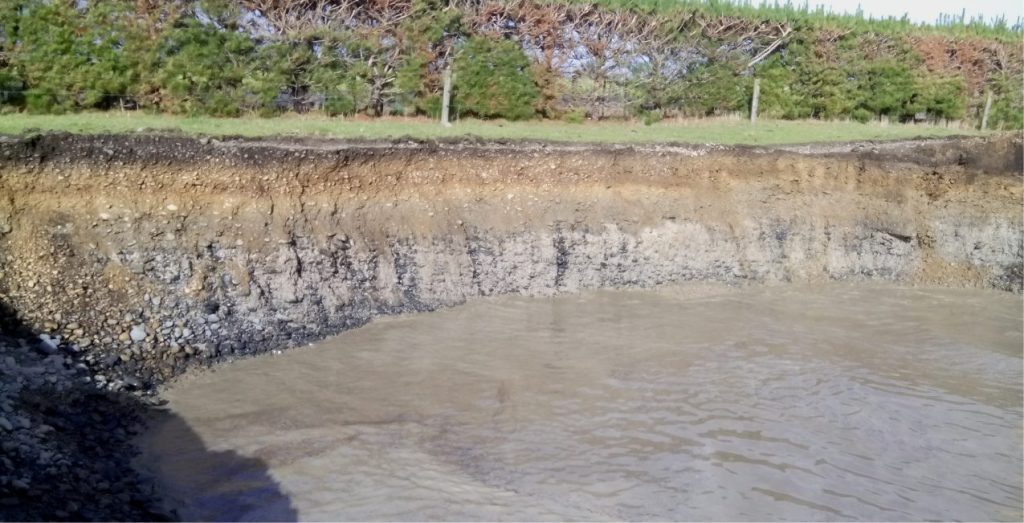
Tabular crossbed lithofacies
Crude tabular crossbedding can form on the landward margins of gravel bars and storm ridges where gravel clasts cascade down steepened slopes.
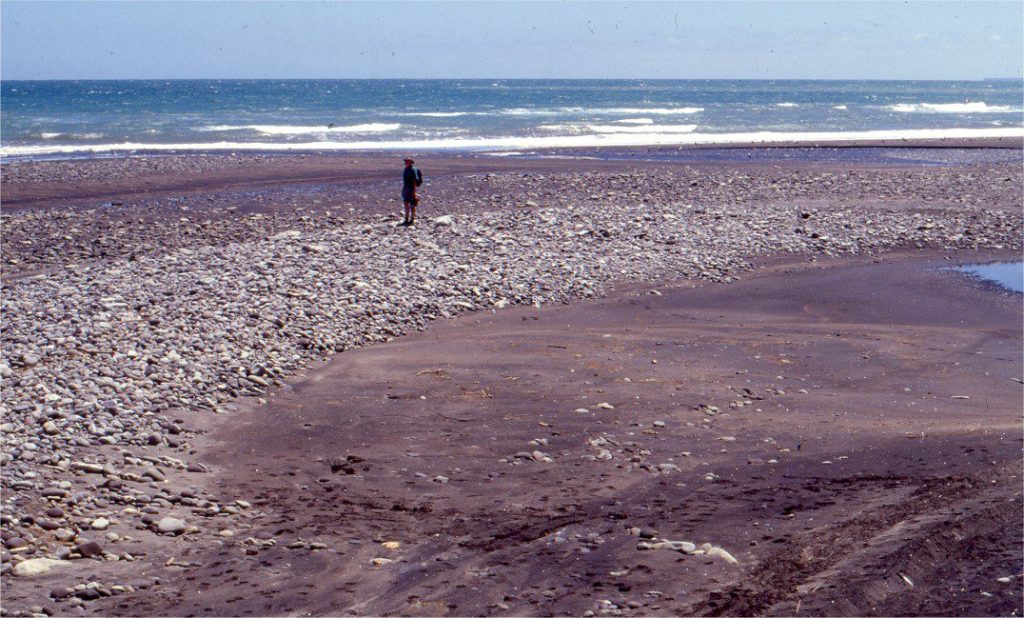
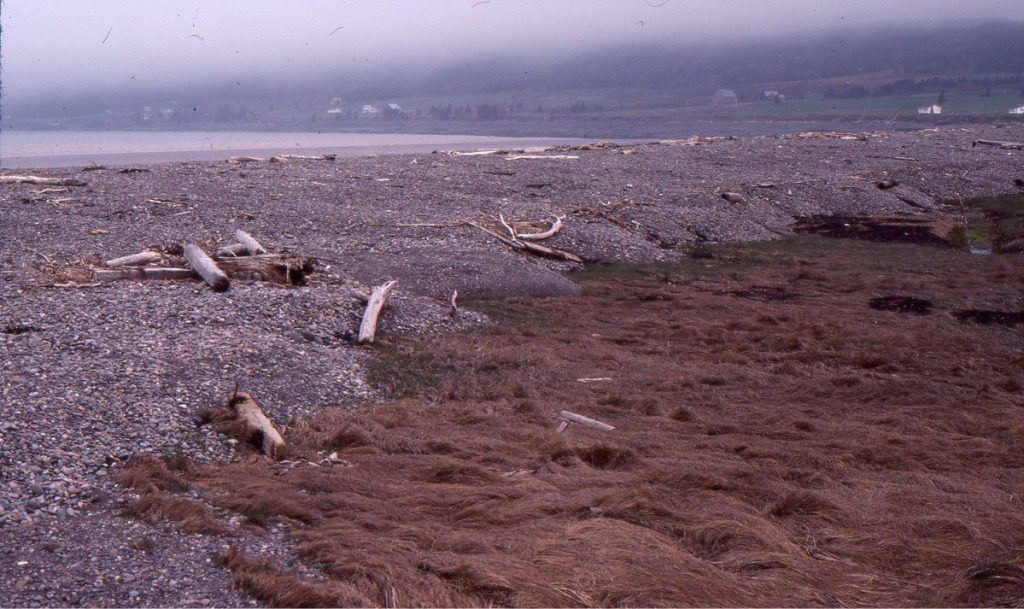
Shoreface gravels
Beach gravels may extend in an unbroken sheet across the shoreface or occur as more isolated deposits separated by sand and gravelly sand. There is a reasonable expectation that gravel clast size will decrease with depth in concert with decreasing wave orbital velocities, although storm waves may completely disrupt this pattern. Palimpsest gravel deposits may also disrupt this trend.
Some of the sedimentary lithofacies encountered in sand-prone shoreface environments will also apply to their gravelly counterparts. Included in this array are tabular, trough, and low-angle crossbed lithofacies, and massive or poorly stratified gravel lithofacies. Hummocky and swaley cross stratification are mostly represented in mixed gravel-sand sediments. Diverse trace fossil assemblages, macro- and microfauna, and in some cases framework biofacies such as corals and bryozoa, can be added to this list. The influence of tides across a shoreface can also be demonstrated if reactivation surfaces, tidal bundles and herringbone crossbeds are present, if not in the gravels, then in the associated sand lithofacies.
The movement of gravel swaths or sheets across a shoreface will also be influenced by changes in relative sea level. Modern and ancient conglomerate packages appear to develop most extensively during progradation. However, episodes of transgression will drive the shoreline landward, and along wave-prone coasts this will be accompanied by the landward transfer of some beach and shoreface gravel. In this case, the expected stratigraphic trends should contain remnant beach and/or shoreface gravels truncated by an erosional ravinement surface that in turn is overlain progressively by a gravel lag and fine-grained, deeper shoreface or outer shelf deposits. A maximum flooding surface will mark the transition to subsequent regression.
Three examples of beach-shoreface stratigraphy are outlined below.
A wave-cut platform
The photo below shows an excellent example of a shore platform eroded into Pliocene mudstone (Tangahoe Formation). The wave-cut platform is a ravinement surface that developed during a Pleistocene interglacial rise in sea level. The platform contains prominent gutters and potholes. It is overlain by semiconsolidated, brown pebble conglomerate and sand (Rapanui Fm.) deposited about 120,000 years ago as the shoreline and shoreface migrated landward. The lower conglomerate, about 1.5 m thick, contains crude subhorizontal and low-angle crossbeds, and a few tabular crossbeds. Larger clasts have been trapped in the gutters, along with shells and wood. The sands higher in the succession contain flaser and lenticular crossbedding indicative of tidal current reversals. The outcrop is located along the north Taranaki coast, New Zealand.
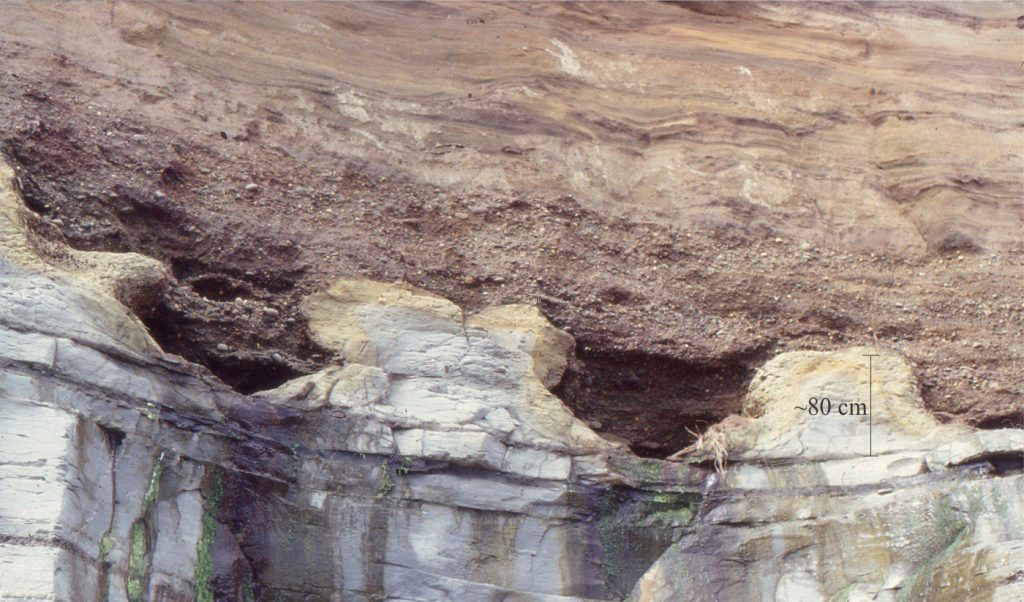
Falher Member, Alberta Basin
Shoreface conglomerate beds are well known in the subsurface and outcrop in Alberta Foreland Basin. One unit, the Albian Falher Member contains several important gas fields and hence has been the subject of much sedimentological scrutiny. Throughout the Falher Member, there are conglomerate lags at transgressive ravinement surfaces, and thicker bedded units of progradational clast and mud-supported pebble conglomerate. For example, the Falher G unit, exposed in the Front Ranges, contains a 12 m thick conglomerate that prograded under relatively high rates of sediment supply, such that the shoreline trajectory moved upward and seaward (Zonneveld, 2004, PDF available). Minor transgressive episodes moved some gravel landward along wave-prone coasts, where the conglomerates overlie ravinement surfaces.
Falher Member conglomerate units are interbedded with sandstone and pebbly sandstone. Together they contain a typical suite of shelf-shoreface trace fossils and sedimentary lithofacies including tabular and trough crossbedding in the conglomerates, and in the sandstones and pebbly sandstones hummocky and swaley crossbedding and current ripples. (Casas and Walker, 1997, PDF available; Zonneveld, 2004).
Waitemata Basin
The Lower Miocene Waitemata Basin (Auckland, New Zealand) is known primarily for its fill of sediment gravity flow deposits (turbidites and debris flows). However, the base of the succession contains a succession of bioclastic sandstone, limestone, and conglomerate (Kawau Formation) deposited in beach and shoreface – shallow shelf environments. The strata drape paleotopographic highs and fill the lows on a Mesozoic-Upper Paleozoic greywacke basement surface. The formation thickness varies from zero to 20-25 m, reflecting the commonly abrupt changes in paleotopography and paleoslope – the paleogeographic picture is one of drowned valleys, resulting in an embayed, rocky coast with numerous pocket beaches, sea stacks and an offshore area dotted with greywacke-cored islands (Ricketts et al.,1989 PDF available). The deposits represent the initial stage of basin subsidence and consequent transgression. They are abruptly overlain by bathyal turbidites, a transition that indicates rapid basin subsidence.
Pocket beaches can be mapped, at some localities, between basement (paleo)sea stacks and rocky promontories. Beach deposits contain pebbles, cobbles and a few boulders of reworked greywacke in massive, subhorizontal and low-angle crossbed lithofacies. The conglomerate lithofacies are interbedded with sandstone beds that contain mostly low-angle crossbedding and some tabular crossbedding. At a few localities there is a demonstrable pinching out of beds where they drape paleotopographic basement highs. Rhodolith concentrations are sometimes nestled against boulders or bedrock protuberances.
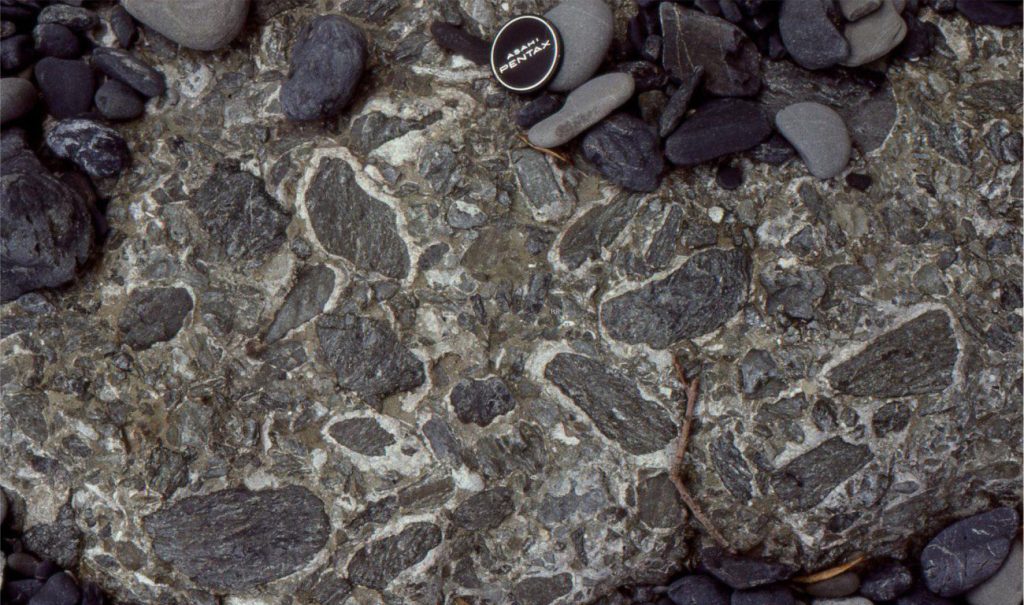
Shoreface conglomerates, sandstones, and bioclastic limestones also contain low-angle crossbedding, and some tabular crossbeds centimetres to a few decimetres thick. Common fossils include barnacles, gastropods, bivalves (including large oysters), brachiopods, solitary corals, bryozoa, rhodoliths, and benthic foraminifera. The shoreface deposits tend to have greater lateral continuity than the pocket beaches because they overlie a more subdued paleotopography.
Trace fossils are common. One form in particular is presented in outcrop cross-sections as vertical-sided, semi-circular scoops 10-20 cm wide, and on bedding as circular to oval structures filled with fine pebbles and sand. In some sections there are 4-6 excavations per linear metre. They are interpreted as ray feeding holes by Murray Gregory et al., (1979).
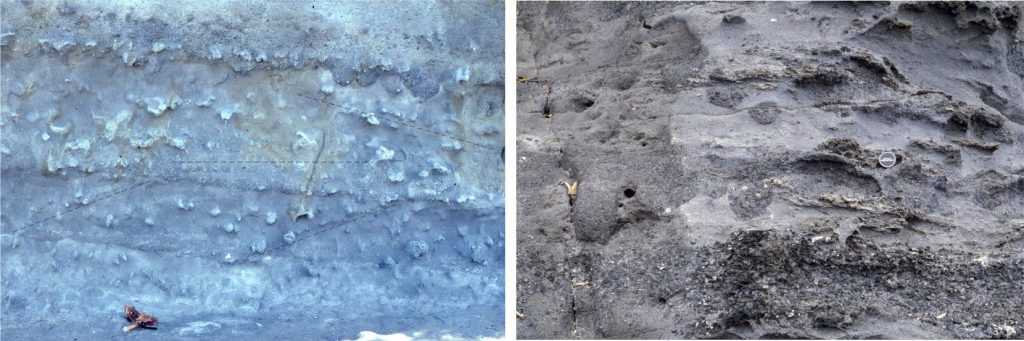
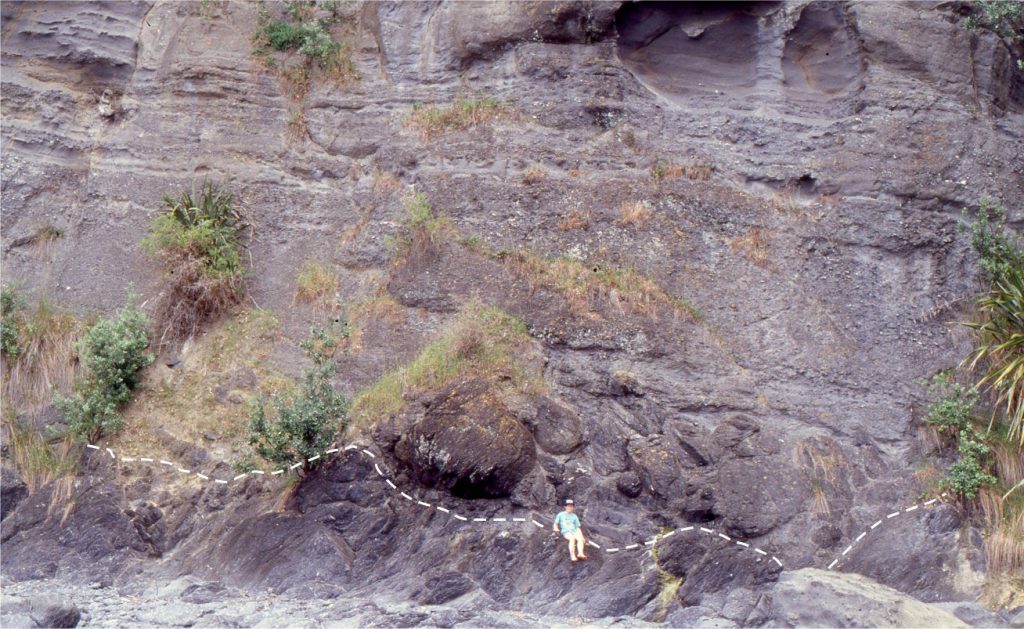
Other posts in this series
Sandstone lithofacies
Sedimentary lithofacies – An introduction
Ripple lithofacies: Ubiquitous bedforms
Ripple lithofacies influenced by tides
Tabular and trough crossbed lithofacies
Laminated sandstone lithofacies
Low-angle crossbedded sandstone
Hummocky and swaley cross-stratification
Lithofacies beyond supercritical antidunes
Subaqueous dunes influenced by tides
Gravel lithofacies
Introducing coarse-grained lithofacies
Crossbedded gravel lithofacies